An official website of the United States government
Official websites use .gov A .gov website belongs to an official government organization in the United States.
Secure .gov websites use HTTPS A lock ( Lock Locked padlock icon ) or https:// means you've safely connected to the .gov website. Share sensitive information only on official, secure websites.
- Publications
- Account settings
- Advanced Search
- Journal List

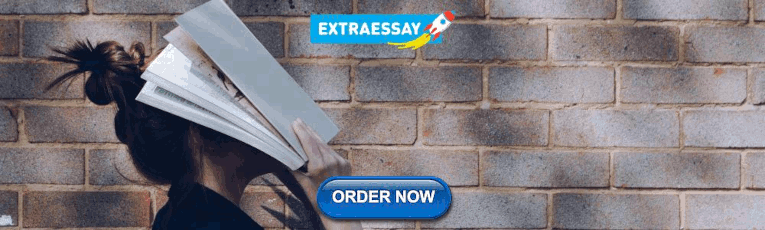
Suppression of experimental autoimmune encephalomyelitis by interleukin-10 transduced neural stem/progenitor cells
Juliane klose, nils ole schmidt, arthur melms, makoto dohi, jun-ichi miyazaki, felix bischof, bernhard greve.
- Author information
- Article notes
- Copyright and License information
Corresponding author.
Contributed equally.
Received 2013 Apr 26; Accepted 2013 Sep 8; Collection date 2013.
This is an open access article distributed under the terms of the Creative Commons Attribution License ( http://creativecommons.org/licenses/by/2.0 ), which permits unrestricted use, distribution, and reproduction in any medium, provided the original work is properly cited.
Neural stem/progenitor cells (NSPCs) have the ability to migrate into the central nervous system (CNS) to replace damaged cells. In inflammatory CNS disease, cytokine transduced neural stem cells may be used as vehicles to specifically reduce inflammation and promote cell replacement. In this study, we used NSPCs overexpressing IL-10, an immunomodulatory cytokine, in an animal model for CNS inflammation and multiple sclerosis (MS). Intravenous injection of IL-10 transduced neural stem/progenitor cells (NSPC IL-10 ) suppressed myelin oligodendrocyte glycoprotein aa 35–55 (MOG35-55)- induced experimental autoimmune encephalomyelitis (EAE) and, following intravenous injection, NSPC IL-10 migrated to peripheral lymphoid organs and into the CNS. NSPC IL-10 suppressed antigen-specific proliferation and proinflammatory cytokine production of lymph node cells obtained from MOG35-55 peptide immunized mice. In this model, IL-10 producing NSPCs act via a peripheral immunosuppressive effect to attenuate EAE.
Keywords: Neural stem/progenitor cells, IL-10, EAE
•Intravenous injected NSPC IL-10 suppress EAE.
•NSPC IL-10 migrate to lymph nodes, spleen and into the CNS.
•NSPC IL-10 inhibit T-cell activation, proliferation and cytokine production.
•This inhibitory effect is independent of indoleamine 2,3-dioxygenase (IDO) and the induction of apoptosis.
Introduction
Multiple sclerosis (MS) is a disabling inflammatory disease of the central nervous system (CNS) characterized by inflammation, demyelination and neurodegeneration [ 1 ]. It is the major disabling disease affecting young individuals. Symptoms such as visual impairment, sensory disturbances and paralysis depend on the location of the lesions in the CNS [ 2 ]. Current approved therapies are immunomodulating and immunosuppressive drugs, including IFN-β, natalizumab, fingolimod, glatiramer acetate, mitoxantrone and teriflunomide.
A promising method for a comprehensive therapy of MS integrating various possible therapeutic mechanisms might be the treatment with neural stem/progenitor cells (NSPCs). Early work demonstrated the ability of neural stem cells to proliferate and differentiate into neurons, oligodendrocytes and astrocytes [ 3 - 5 ], and to display an inherent tropism for neuropathology [ 6 ]. It has been shown in experimental autoimmune encephalomyelitis (EAE), the animal model of MS, that transplantation of neural stem cells promotes remyelination and reduces brain inflammation [ 7 , 8 ].
NSPCs have been transplanted into recipient animals to replace injured or damaged neural tissue. This has been evaluated in various models of degenerative and inflammatory CNS diseases [ 9 ]. Neural stem cells migrate into areas of neuropathology such as malignant glioma [ 10 ], brain injury [ 11 ] and inflamed CNS tissue during EAE [ 8 , 12 ]. Moreover, neural stem cells have been shown to be able to inhibit inflammation within the CNS in EAE immunized mice [ 13 ].
Taking into account this unique pathotropism, we assumed that NSPCs could be used as a cell-based delivery model for immunomodulatory molecules. We choose to use IL-10 as an immunomodulatory cytokine to be delivered by NSPCs into the CNS of mice immunized to develop EAE.
IL-10, a regulatory cytokine mainly produced by T-helper type 2 (Th2) cells, inhibits the activity of Th1 and Th17 cells, the production of proinflammatory cytokines, including TNFα, IL-1 and IFN-γ, and chemokines, as well as the expression of major histocompatibility complex (MHC) class II molecules by antigen-presenting cells (APCs) [ 14 - 16 ]. IL-10 also inhibits macrophages and monocytes, and enhances antibody production and survival of B-lymphocytes [ 16 ]. IL-10-deficient mice develop more severe EAE than wild-type mice, overexpression of IL-10 protects mice from EAE [ 17 ] and subcutaneous or intranasal treatment reduces disease severity [ 18 ]. Thus, IL-10 is an efficient anti-inflammatory cytokine which potently suppresses EAE and is therefore a suitable candidate molecule to be used in our approach. In addition, the immunomodulatory capacity of IFN-β treatment in patients with MS is mediated at least in part by induction of IL-10 production by immune cells.
In this study, we demonstrate that the intravenous injection of IL-10 producing NSPCs in myelin oligodendrocyte glycoprotein aa 35–55 (MOG35-55) peptide immunized C57BL/6 mice during the initial phase of EAE resulted in an attenuated disease course. NSPCs migrated to peripheral lymphoid organs as well as into the CNS, and inhibited proliferation and production of proinflammatory cytokines by autoreactive T-cells. Intravenous injection of IL-10 producing NSPCs attenuates EAE via a peripheral immunosuppressive effect potentially via immunosuppression within the CNS.
Materials and methods
Murine nspcs.
NSPCs were established from the frontoparietal brains of 2-week-old C57BL/6 mice and characterized as previously described [ 19 ]. NSPC IL-10 was established by retroviral transduction with pMSCV-IL10 using a commercially available kit (MSCV Retroviral Expression System, BD Biosciences, San Jose, CA, USA).
The cells were grown as neurospheres in complete NSPC growth medium containing neurobasal medium (Gibco, Karlsruhe, Germany) supplemented with 100 μg/mL penicillin/streptomycin (Gibco), 10 mM L-glutamine (Gibco), 20 μL/mL B-27 supplement (Gibco) and 0.25 μg/mL fungizone (Gibco). Recombinant epidermal growth factor (EGF) (20 ng/mL, PeproTech, London, UK), recombinant basic fibroblast growth factor (FGF) (20 ng/mL, PeproTech) and heparin (1000 IE, Braun, Melsungen, Germany) were added directly to the tissue culture flasks twice a week. NSPCs were routinely harvested and mechanically dissociated into a single cell suspension when the neurospheres reached 200 to 500 mm. Single cell suspensions were used for further passages, intravenous injections and in vitro experiments. Supernatants were collected and stored at −80°C for further experiments.
Animals, EAE induction and NSPC injection
Female C57BL/6 mice were obtained from Charles River (Sulzfeld, Germany) and housed under pathogen-free conditions at the animal facility of the Hertie-Institute for Clinical Brain Research (Tübingen, Germany). All experiments were carried out in accordance with local regulations and approval was obtained in advance (Regierungspräsidium Tübingen, animal experimentation protocol TV N9/04 to BG). At the age of 5 to 6 weeks, mice were immunized with 60 μg MOG35-55, dissolved in 100 μL PBS (PAA Laboratories, Pasching, Austria) and emulsified with 100 μL incomplete Freund’s adjuvant (IFA) (Sigma-Aldrich, Steinheim, Germany) containing 400 μg Mycobacterium tuberculosis (Difco Laboratories, Detroit, MI, USA). On the day of immunization and 2 days after immunization, 150 ng Bordetella pertussis toxin (Merck, Darmstadt, Germany) was injected intravenously. NSPC IL-10 , NSPCs or PBS as a negative control was injected intravenously on day 7 post-immunization, or on first sign of disease (1 × 10 6 cells per injection).
For immunization of 2D2 mice, female 2D2 TCR transgenic mice were obtained from Dr Bettelli [ 20 ] and housed under specific pathogen-free conditions. Mice aged 5 to 6 weeks were immunized with 25 μg MOG35-55 dissolved in 100 μL PBS and emulsified with 100 μL IFA containing 400 μg M tuberculosis . On day 0 and day 2 pi, 150 ng B pertussis toxin was injected intravenously. At day 5 post-immunization, 1 × 10 6 NSPC IL10 , NSPCs or PBS was injected intravenously. As a result of the MOG antigen-specific TCR, 2D2 transgenic mice are more sensitive to MOG-specific immunization. Therefore, only a concentration of 25 μg MOG35-55 was used for immunization. At 14 days post-immunization, cells were isolated from draining lymph nodes and cultured in RPMI 1640 medium containing 5 μg/mL or 50 μg/mL MOG35-55 peptide. Proliferation was determined after 72 hours by 3 H-thymidine incorporation as previously described [ 21 ]. Cytokine concentrations in culture supernatants were measured after 48 hours by enzyme-linked immunosorbent assay (ELISA) (eBioscience, San Diego, CA, USA).
Animals were monitored daily starting at least at day 5 post-immunization and clinical signs scored as follows: 0, no paralysis; 1, limp tail; 2, limp tail and weak gait; 3, hind limb paralysis; 4, fore limb paralysis; and 5, death.
Prior to injection, NSPCs were labeled with 4 × 10 6 molar PKH26 dye for 5 minutes at room temperature. Dye reaction was stopped with RPMI 1640 medium containing FBS; cells were washed and injected as previously described. Two weeks after immunization, brain tissue and spinal cord were isolated, fixed with 4% paraformaldehyde (PFA) for 24 hours, incubated for 24 hours in 20 sucrose and frozen in liquid nitrogen. Spleen, lymph nodes, liver and lungs were immediately frozen in liquid nitrogen. Frozen sections were stained with mounting medium containing DAPI (Linaris, Wertheim, Germany) and analyzed for PKH26-labeled cells by fluorescence microscopy. In addition, brain sections were stained with hematoxylin and eosin (H&E), and analyzed by microscopy.
Spleen cell cultures
Spleens from naive 2D2 TCR transgenic mice and C57BL/6 mice were isolated and cultured with RPMI 1640 medium containing 0.5 μg/mL, 5 μg/mL or 50 μg/mL MOG35-55 peptide, or 0.5 μg/mL or 1 μg/mL concanavalin A (ConA) in the presence of NSPC IL-10 or NSPC culture supernatants. To assess effects of NSPC co-cultivation, isolated naive 2D2 or C57BL/6 spleen cells were cultured with NSPC IL-10 or NSPCs at a NSPC/spleen cell ratio of 1:1, 1:10 or 1:100 in RPMI 1640 medium containing 5 μg/mL MOG35-55 or 1 μg/ml ConA. Proliferation after 72 hours was detected by a 3 H-thymidine incorporation assay. Supernatants were collected after 48 hours, and IL-17, IL-2 and IFN-γ concentrations were measured by ELISA. Neurobasal medium served as a control.
Cytokine concentrations were measured by ELISA according to the manufacturer’s instructions (IL-2, IL-10 and IFN-γ, BD Biosciences; IL-17, eBioscience). ELISA plates (NUNC, Kamstrupvej, Denmark) were coated overnight with capture antibody diluted in coating buffer (0.2 M sodium phosphate, pH 6.5). After 1 hour of blocking with assay diluent (PBS containing 10% FBS), cytokine-containing supernatants (eventually diluted with assay diluent) were incubated for 2 hours, followed by 1 hour of incubation with biotinylated secondary antibody and streptavidin-peroxidase. Plates were developed using 3,3’5,5’-tetramethylbenzidine (TMB) (Sigma-Aldrich). Reaction was stopped with 1 M hydrogen chloride and plates were measured at 450 nm using the microplate reader Multiskan Ex (Thermo, Waltham, MA, USA). Standard curves were calculated based on measurements of different concentrations of recombinant cytokines. Plates were washed with PBS containing 0.02% Tween 20 (Roth, Karlsruhe, Germany). All steps were performed at room temperature, except for the coating (4°C).
The IL-10 enzyme-linked immunosorbent spot (ELISPOT) was performed using a commercially available kit (Human IL-10 ELISPOT Ready-SET-Go!, eBioscience). Multiscreen TM-HA plates (Millipore, Billerica, MA, USA) were coated overnight with coating antibody diluted in coating buffer. After 1 hour of blocking with RPMI 1640 medium, 4 × 10 5 NSPC IL-10 or NSPCs were incubated at 37°C, 5% CO 2 for 48 hours. Plates were incubated with biotinylated secondary antibody for 2 hours and subsequently with streptavidin-peroxidase for 45 minutes, and then developed with AEC substrate solution (0.1 M acetate solution, pH 5.0) for 60 minutes. Resulting spots were analyzed with an ELISPOT reader. At every step, the plates were washed with PBS containing 0.02% Tween 20. All steps were performed at room temperature, except for the coating with the capture antibody (4°C).
Total RNA was isolated from spleen cells of naive C57BL/6 mice or from cultured NSPC IL-10 and NSPCs (using a kit from Seqlab, Göttingen, Germany). RNA was transcribed into cDNA using products from Promega (Madison, WI, USA). PCR reactions were performed in a thermocycler (Biozym, Oldendorf, Germany) using the following conditions: 35 cycles for 1 minute at 94°C, 2 minutes at 55°C and 3 minutes at 72°C. Reaction was completed by incubation at 72°C for 10 minutes. PCR products were separated by gel electrophoresis using 2% agarose gels containing ethidium bromide. PCR primers specific for mouse indoleamine 2,3-dioxygenase (IDO) were 5’GCCCACCGGAACTTCCTTT3’ (forward) and 5’CACTCGTTATAAGCTTTCGTCAAGTC 3’ (reverse). Amplification of β-actin served as a control. PCR primers for mouse β-actin were 5’TGTCCACCTTCCAGCAGATGT 3’ (forward) and 5’AGCTCAGTAACAGTCCGCCTAGA 3’ (reverse) [ 22 ].
IDO inhibition assays
1-methyl-DL-tryptophan (1-MT) (Sigma-Aldrich) was used as an IDO inhibitor. Isolated 2D2 spleen cells were cultured in RPMI 1640 medium containing 5 μg/mL MOG35-55 and NSPC IL-10 or NSPCs (NSPC/spleen cell ratio of 1:1) in the presence or absence of 500 μg/mL 1-MT. Proliferation after 72 hours was detected by 3 H-thymidine incorporation.
Statistical analysis
EAE experiments with NSPC injection on day 7 after EAE induction were performed with 15 mice per group and EAE experiments with NSPC injection after onset of clinical symptoms with seven mice per group. Proliferation, ELISA and flow cytometry experiments were carried out in triplicate. Values are expressed as mean ± SD. Statistical analysis was performed with SPSS Statistics 17.0 for Windows (IBM Armonk, NY, USA). For the EAE experiments, a survival analysis and analysis of variance (ANOVA) with a post-hoc Tukey-Kramer test were performed. In vitro experiments were analyzed using ANOVA with post-hoc Bonferroni testing.
NSPC culture
NSPCs were cultured in the presence of basic FGF and EGF from neurospheres (Figure 1 a). As expected, phenotypic characterization by flow cytometry showed multipotency of NSPCs and a percentage of neuronal stem cells among total NSPCs of around 5% (O4 - PSA-NCAM - CD81 - Prominin-1) [ 19 , 23 ]. IL-10 production of transduced cells was confirmed using an IL-10 ELISPOT assay and an IL-10 ELISA (Figure 1 b,c). NSPCs and NSPC IL-10 were indistinguishable with regard to expression of differentiation markers (90% expressed CD81, <10% expressed O4 and PSA-NCAM, 10% expressed CD133, and 20% expressed A2B5 (Figure 1 d).
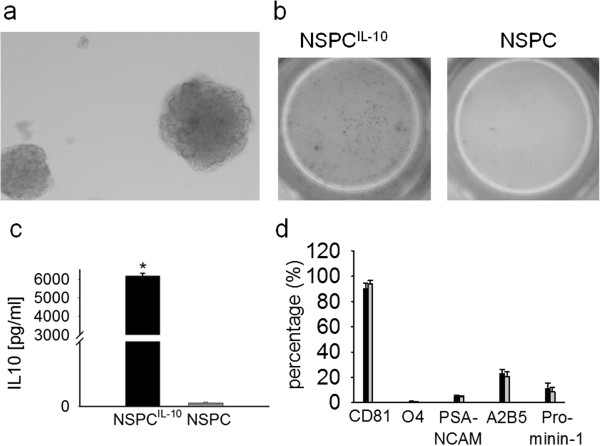
NSPC and IL-10 production. (a) Morphology of neurospheres as demonstrated by a phase contrast image of a representative neurosphere culture in presence of 20 ng/mL FGF-2 and 20 ng/mL EGF. (b) IL-10 production of NSPC IL-10 and NSPCs was determined by IL-10 ELISPOT assay (dots indicating IL-10 producing cells), and (c) IL-10 ELISA. * t -test, P <0.001. (d) Analysis of NSPC IL-10 (black bar) and NSPCs (grey bar) for expression of different stem cell markers by flow cytometry. EGF, epidermal growth factor; ELISA, enzyme-linked immunosorbent assay; ELISPOT, enzyme-linked immunosorbent spot; FGF, fibroblast growth factor; IL, interleukin; NSPC, neural stem/progenitor cell; PSA-NCAM, polysialic acid neural cell adhesion molecule.
Injected NSPC IL-10 suppress EAE when injected during peripheral T-cell activation
To analyze the effect of NSPC IL-10 on the development of CNS-directed autoimmunity, 1 × 10 6 NSPC IL-10 was injected intravenously into C57BL/6 mice on day 7 after EAE induction with MOG35-55 peptide. Disease severity and maximum EAE disease score were reduced in mice that received NSPC IL-10 compared to NSPC- or PBS-treated control animals. The mean maximum disease score in NSPC IL-10 -treated mice was significantly reduced in comparison to NSPC-treated mice or PBS-treated control animals ( P = 0.02). Furthermore, NSPC IL-10 -treated mice showed a milder EAE disease score during the whole observation period and the lowest sum score in comparison with control groups (Figure 2 a). The effects of NSPC IL-10 injection after disease onset were also examined. Injection of 1 × 10 6 NSPC IL-10 into MOG35-55 immunized C57BL/6 mice 1 day after the beginning of clinical EAE only mildly influenced the disease course (Figure 2 a).
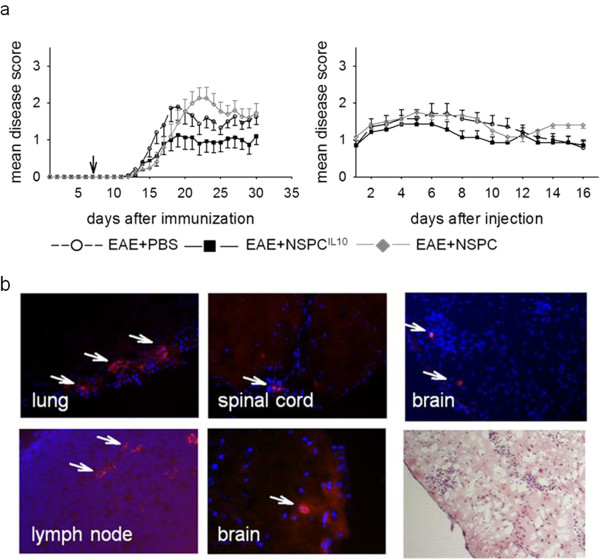
NSPC IL-10 suppress clinical scores in EAE. EAE was induced in C57BL/6 mice by subcutaneous injection of 50 μg MOG35-55 peptide in CFA plus intravenous injection of 150 ng Bordetella pertussis toxin. (a) On day 7 after EAE induction or (a) after established EAE disease, mice were intravenously injected with 1 x 10 6 NSPC IL-10 , NSPCs or PBS as indicated. (b) 1 x 10 6 PKH26-labeled NSPC IL-10 , NSPCs or PBS were injected 7 days after induction of EAE in 2D2 mice with MOG35-55. On day 7 after injection, organs were examined by fluorescence microscopy for the presence of PKH26 + cells. Cell nuclei were stained with DAPI (blue). Infiltration was analyzed by H&E stain. NSPC IL-10 and NSPCs were detected within lungs, spinal cords, lymph nodes and brains of MOG35-55 immunized 2D2 mice and in the CNS within cellular infiltrates. Tissues are shown from NSPC IL-10 -treated mice as representative examples for NSPC- and NSPC IL-10 -treated mice. CFA, complete Freund’s adjuvant; CNS, central nervous system; DAPI, 4’,6-diamidino-2-phenylindole; EAE, experimental autoimmune encephalomyelitis; H&E, hematoxylin and eosin; IL, interleukin; MOG35-55, myelin oligodendrocyte glycoprotein aa 35–55; NSPC, neural stem/progenitor cell; PBS, phosphate-buffered saline.
In order to assess how NSPC IL-10 exerts suppressive effects in vivo , we determined their migratory properties after intravenous injection into immunized animals. Mice with MOG35-55-induced EAE received intravenous injections of NSPC IL-10 and NSPCs labeled with the fluorescent dye PKH26. Two weeks after injection, PKH26 + NSPC IL-10 and NSPCs were present in lungs, lymph nodes and inflammatory infiltrates within the CNS (Figure 2 b), while no PKH26-labeled NSPC IL-10 or NSPCs could be detected within the liver.
NSPC IL-10 suppress T-cell proliferation and cytokine production in draining lymph nodes
MOG35-55-induced EAE is a T-cell-mediated disease, and autoreactive T-cells in EAE are activated within peripheral lymph nodes before they migrate into the CNS [ 24 ]. Since intravenously injected NSPC IL-10 migrate to peripheral lymph nodes, we considered whether NSPC IL-10 influence the activity or function of autoreactive T-cells within this compartment. To this end, C57BL/6 mice carrying a transgenic TCR specific for MOG35-55 (2D2 mice) [ 20 ] were immunized with MOG35-55 and treated 7 days following immunization with NSPC IL-10 or PBS. Draining lymph node cells were isolated 14 days post-immunization, and assessed for proliferation and cytokine production upon stimulation with MOG35-55 peptide. Treatment with NSPC IL-10 reduced the proliferative capacity of autoreactive T-cells in draining lymph nodes (Figure 3 a) in this transgenic model. In addition, the capacity to produce IFN-γ was reduced in treated animals; IL-17 and IL-10 were only detected at low levels under these experimental conditions (Figure 3 b). Transferred NSPC IL-10 thus inhibited proliferation and IFN-γ production by autoreactive T-cells at the site of peripheral T-cell activation in vivo .
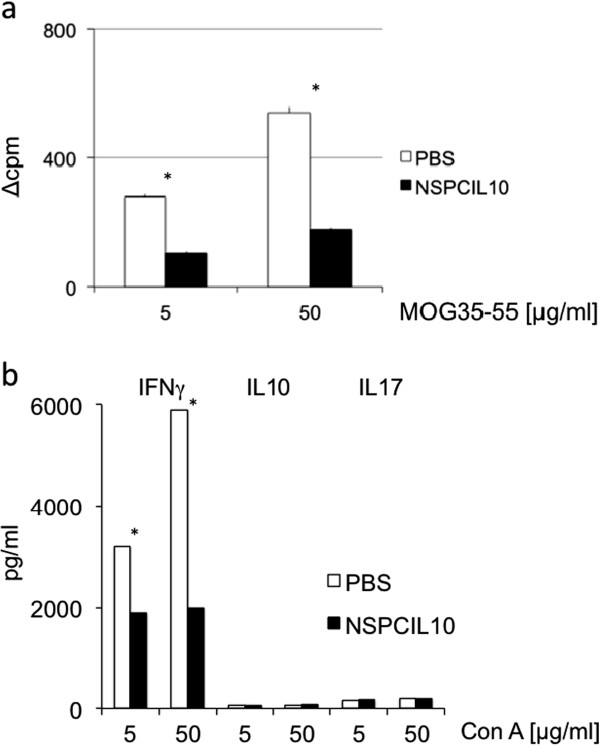
NSPC IL-10 cells reduce proliferation and IFN-γ production by lymph node cells. 2D2 mice with MOG35-55-induced EAE received intravenous injections of 1 x 10 6 NSPC IL-10 , NSPCs or PBS on day 7 after disease induction. Draining lymph node cells were isolated 7 days after injection and stimulated with MOG35-55 peptide. (a) Proliferation was determined by 3 H-thymidine incorporation, and (b) IFN-γ, IL-10 and IL-17 production was determined by ELISA. * P <0.05. NSPC IL-10 versus NSPCs are expressed as mean values with SD from three independent experiments. ConA, concanavalin A; EAE, experimental autoimmune encephalomyelitis; ELISA, enzyme-linked immunosorbent assay; IFN, interferon; IL, interleukin; MOG35-55, myelin oligodendrocyte glycoprotein aa 35–55; NSPC, neural stem/progenitor cell; PBS, phosphate-buffered saline.
Immunosuppressive effects of NSPC IL-10 and NSPC IL-10 supernatant in vitro
To further investigate the immunosuppressive effects of NSPC IL-10 , we cultivated NSPC IL-10 with MOG35-55-activated spleen cells isolated from naive 2D2 mice at different cell ratios. MOG35-55-activated spleen cells cultivated with NSPCs or neurobasal medium served as controls. NSPC IL-10 suppressed proliferation of MOG35-55-activated cells as determined by 3 H-thymidine incorporation, and production of IL-2 and IFN-γ (Figure 4 a). A co-culture ratio of NSPC IL-10 /spleen cells of 1:1 lead to maximal inhibition of proliferation and cytokine production, and a NSPC IL-10 /spleen cell ratio of 1:100 still suppressed proliferation and cytokine production.
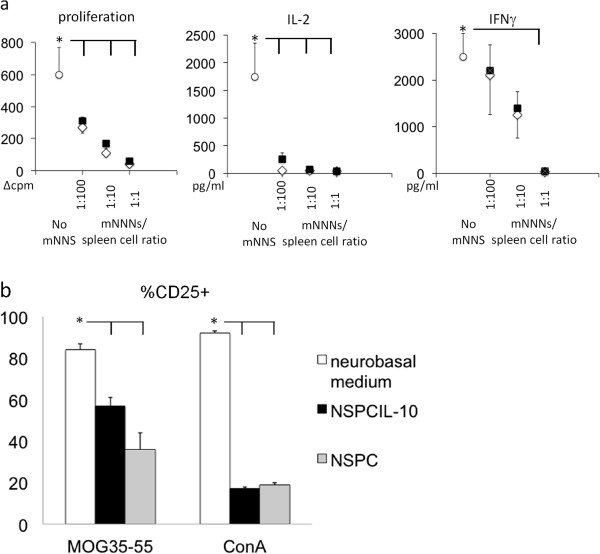
NSPC IL-10 and NSPCs inhibit proliferation and cytokine production by T-cells. (a) C57BL/6 spleen cells were polyclonal-activated with ConA in the presence of NSPC IL-10 (black squares), NSPCs (open diamonds) or neurobasal medium (open circles) as indicated. Proliferation was determined by 3 H-thymidine incorporation. IFN-γ and IL-2 production was assessed by ELISA. * P <0.05. Mean values are expressed with SD from three independent experiments. (b) Frequency of CD25 + cells of CD4 T-cells of MOG35-55-stimulated 2D2 spleen cells or ConA-stimulated spleens cells after cultivation with NSPC IL-10 , NSPCs or neurobasal medium. Analysis was performed by flow cytometry. * P <0.05. NSPC, neural stem/progenitor cell; ConA, concanavalin A; ELISA enzyme-linked immunosorbent assay; IFN, interferon; IL, interleukin; MOG35-55, myelin oligodendrocyte glycoprotein aa 35–55.
To assess whether NSPCs and NSPC IL-10 influence T-cell activation, we determined the expression of the IL-2 receptor α chain (CD25) on antigen-specific and polyclonal stimulation of spleen cells by flow cytometry. MOG35-55 or ConA-stimulated spleen cells were cultivated in the presence of NSPC IL-10 , NSPCs or neurobasal medium. NSPCs and NSPC IL-10 potently suppressed activation of CD4 T-cells as determined by CD25 expression (Figure 4 b).
To evaluate whether immunosuppression by NSPCs and NSPC IL-10 is mediated by soluble factors, culture supernatants of NSPCs and NSPC IL-10 were collected and incubated with antigen-specific or polyclonal stimulation of spleen cells. Culture supernatants of NSPCs and NSPC IL-10 inhibited proliferation, and IFN-γ and IL-17 production of ConA-stimulated cells in comparison to neurobasal medium (Figure 5 a,b,c).
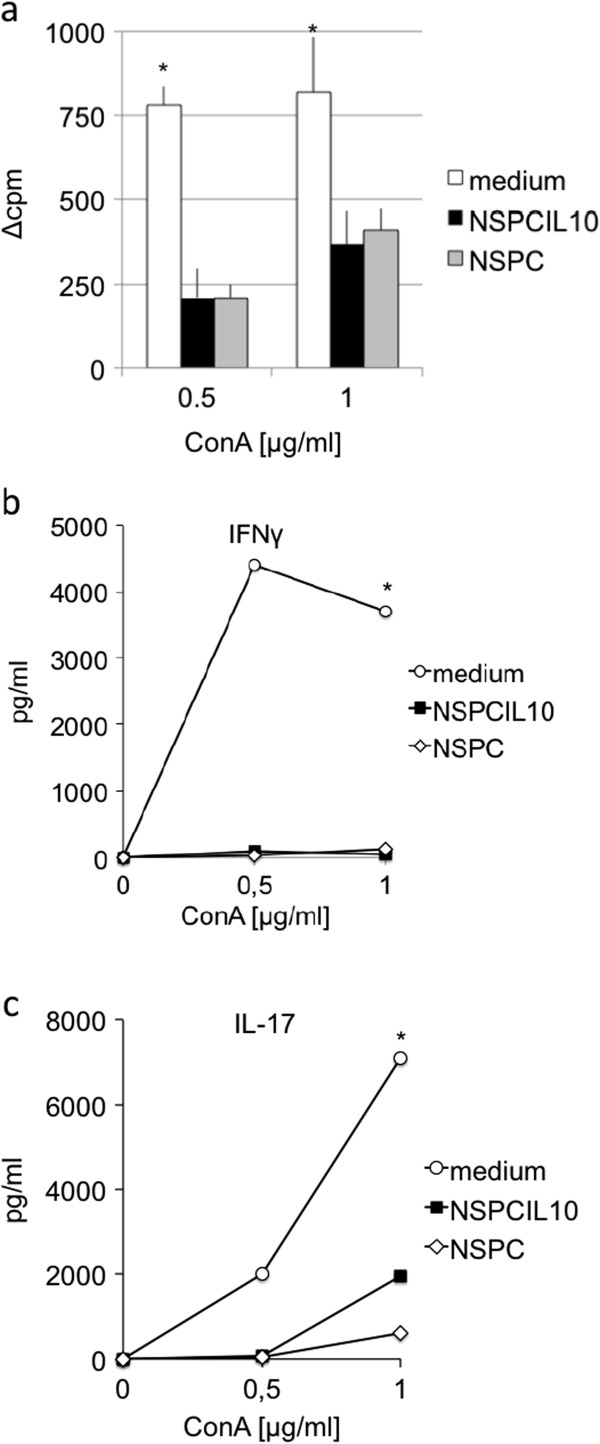
Supernatant of NSPC IL-10 inhibits proliferation, and the production of IFN-γ and IL-17 by spleen cells. C57BL/6 spleen cells were stimulated with ConA in the presence of neurobasal medium (white bar, circles) or culture supernatant of NSPC IL-10 (black bar, squares) or NSPC (grey bar, diamonds). (a) Proliferation was determined by 3 H-thymidine incorporation, and production of (b) IFN-γ and (c) IL-17 was assessed by ELISA. * P <0.05. Mean values are expressed with SD from three independent experiments. ConA, concanavalin A; ELISA, enzyme-linked immunosorbent assay; IFN, interferon; IL, interleukin; NSPC, neural stem/progenitor cell.
Inhibition of T-cell proliferation by NSPC IL-10 is not mediated by IDO or induction of apoptosis
NSPC IL-10 inhibited T-cell proliferation. T-cell proliferation depends on the availability of tryptophan, which is closely regulated by the tryptophan-degrading enzyme IDO [ 25 ]. IDO expression is induced in many cell types, including macrophages, dendritic cells, fibroblasts, microglia and astrocytes by the proinflammatory cytokines IFN-γ and IFN-β. We considered whether immunosuppression by NSPC IL-10 is related to IDO activity. In vitro cultivated NSPC IL-10 and NSPCs expressed large amounts of IDO as demonstrated by RT-PCR (Figure 6 a). Inhibition of IDO by the tryptophan analogue 1-MT [ 26 ] did not alter the ability of NSPC IL-10 to inhibit polyclonal T-cell proliferation, indicating that immunosuppression by NSPC IL-10 is not mediated by IDO (Figure 6 b).
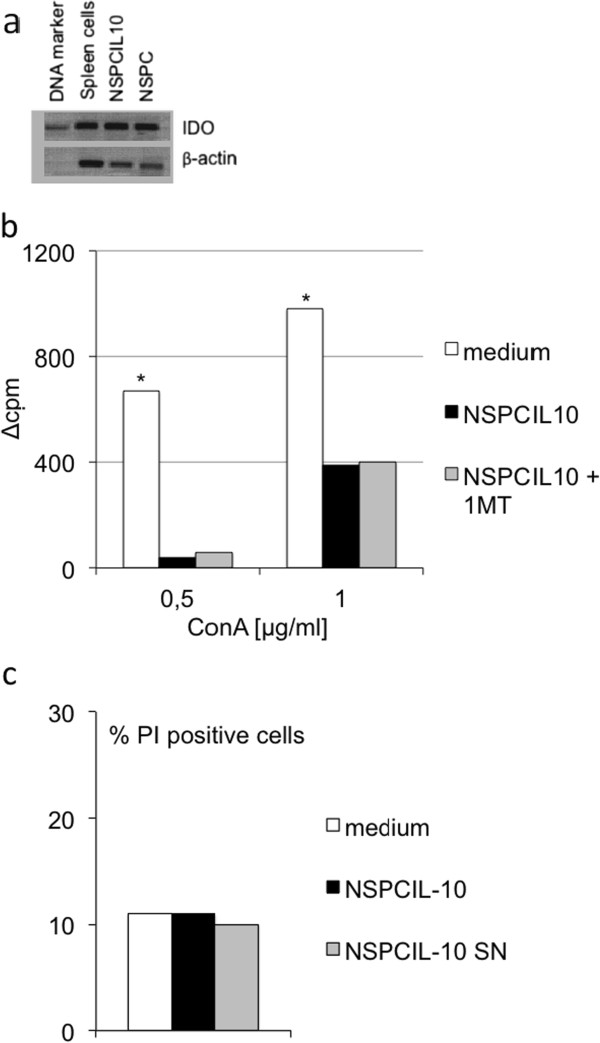
Immunosuppression of NSPC IL-10 is not mediated by IDO and not due to induction of apoptosis. (a) NSPC IL-10 and NSPCs express IDO. IDO expression was detected by RT-PCR in spleens, NSPC IL-10 and NSPCs. (b) Inhibition of IDO by 1-MT does not abrogate the immunosuppression mediated by co-culture of NSPC IL-10 . C57BL/6 spleen cells were activated with ConA in the presence of neurobasal medium, NSPC IL-10 or NSPC IL-10 and 1-MT. Proliferation was determined by 3 H-thymidine incorporation. (c) NSPC IL-10 and NSPC IL-10 culture supernatants do not induce apoptosis in activated spleen cells. C57BL/6 spleen cells were stimulated with ConA in the presence of medium, NSPC IL-10 and NSPC IL-10 culture supernatant. After 24 hours, the frequency of PI + cells was determined by flow cytometry. 1-MT, 1-methyl-DL-tryptophan; ConA, concanavalin A; IDO, indoleamine 2,3-dioxygenase; IL, interleukin; NSPC, neural stem/progenitor cell; PI, propidium iodide; RT-PCR, reverse transcription polymerase chain reaction.
We also examined whether NSPC IL-10 mediated immunosuppression by induction of apoptotic cell death. We found no enhanced induction of apoptotic cell death in polyclonal stimulation of spleen cells after cultivation with NSPC IL-10 or NSPC IL-10 culture supernatants (Figure 6 c). We conclude that the immunosuppressive effects of NSPC IL-10 are not due to enhanced induction of apoptosis in CNS-specific or polyclonal T-cells.
Different sources of somatic stem cells, including neural progenitor cells [ 8 , 27 ], hematopoietic [ 28 , 29 ] and mesenchymal stem cells [ 30 - 32 ], are currently explored for their immunomodulatory capacity in trauma, stroke, neurodegenerative diseases and CNS-directed autoimmunity. NSPCs have the unique ability to migrate directly into the damaged brain [ 6 , 27 , 33 , 34 ], and cytokines released by activated cells during the disease process act as chemoattractants for NSPCs [ 11 , 35 - 37 ]. We made use of the specific tropism of neural stem cells to deliver IL-10 into sites of inflammation and neurodegeneration in an animal model of CNS inflammation. To this end, we injected IL-10 producing NSPCs in MOG35-55 peptide immunized mice and observed EAE disease severity, migration pattern and immune reactions to evaluate the therapeutic potential of IL-10 producing NSPCs.
Injection of NSPC IL-10 at the time of peripheral T-cell activation induced a robust reduction of disease severity, while injection after onset of clinical symptoms did not significantly alter the disease course. After intravenous injection, NSPCs and NSPC IL-10 migrated into the CNS, as well as into peripheral lymph nodes and spleen. These results indicate that NSPCs are suitable vectors for drug delivery into the diseased CNS but also migrate to peripheral lymphoid organs. This is consistent with earlier studies using neural precursor cells [ 38 ].
We analyzed the immune reaction defined by proliferation and cytokine expression of lymph node cells from MOG35-55 immunized 2D2 mice treated with NSPC IL-10 . NSPC IL-10 suppressed ex vivo proliferation of T-cells isolated during the peak of the disease. Moreover, NSPC IL-10 suppressed production of IFN-γ but not IL-10 by MOG35-55-specific T-cells, indicating suppression of Th1-related cytokines. During the induction phase of EAE, encephalitogenic T-cells migrate from draining lymph nodes to the spleen and subsequently into the CNS [ 39 ]. Collectively, these results indicate that intravenously injected NSPC IL-10 suppress EAE by migrating into peripheral lymphoid organs where they inhibit T-cell proliferation and cytokine production.
This immunosuppressive effect of NSPC IL-10 on immune cells was confirmed in vitro . NSPC IL-10 suppressed proliferation and IL-2 and IFN-γ production by antigen-specific and polyclonal stimulation of spleen cells, and the presence of NSPC IL-10 cells also inhibited expression of IL2Rα demonstrating reduced T-cell activation. This inhibitory effect is mediated at least in part by direct interaction of NSPC IL-10 and T-cells as it was also observed after mitogen activation of T-cells in the absence of APCs. In addition, IL-10 could reduce inflammation by reducing MHC class II expression by APCs.
Other studies have shown similar peripheral immunosuppressive effects after peripheral injection of neural stem cells or neural precursor cells. In these studies, intravenously injected neural precursor cells migrated to peripheral lymphoid organs and inhibited proliferation, activation or release of proinflammatory cytokines by autoreactive T-cells [ 7 ]. Subcutaneously injected neural stem cells migrated into lymph nodes and inhibited the generation of effector T-cells [ 38 ]. In our experiments, disease suppression was maximal when NSPC IL-10 was injected at the time of peripheral T-cell activation. This observation further supports the notion that NSPC IL-10 attenuates EAE by inhibiting T-cell activation in peripheral lymphoid organs rather than within the CNS.
Non-transfected NSPCs were used as a control in all experiments. Injection of NSPCs at the time of peripheral T-cell activation did not result in reduction of clinical EAE as opposed to injection of NSPC IL-10 . Other studies have previously shown beneficial effects of neural stem cells or neural precursor cells on EAE disease course [ 7 , 27 , 33 , 38 ]. Differences in EAE models, mouse-strains, NSPC-types or time points of injection could contribute to these different results. In this study, the migration pattern of NSPCs was similar compared to the pattern of NSPC IL-10 and also comparable to previous observations. We detected NSPCs in peripheral lymphoid organs and within the CNS after intravenous injection similar to our results with NSPC IL-10 . We also found an inhibitory effect on proliferation and IL-2/IFN-γ production of antigen-specific activated lymph node cells and spleen cells isolated after 35 days pi, and during the disease peak. Moreover, NSPCs inhibited proliferation and IL-2/IFN-γ production of antigen-specific and polyclonal stimulation of spleen cells in co-cultivation experiments. Thus, we were able to replicate in vitro effects of neural stem cells or NSPCs described previously. However, in contrast to previous studies, we were not able to show in vivo effects of non-transfected NSPCs. In general, the inhibitory in vitro effects of NSPCs were weaker than those of NSPC IL-10 .
Another possible mechanism by which NSPCs may inhibit immune cells could be the induction of apoptosis. Previous studies have shown that mesenchymal stem cells [ 40 ] and neural multipotent precursor cells [ 8 ] induced apoptosis in activated T-cells. In addition, Yang et al . [ 13 ] demonstrated that IL-10-expressing neural stem cells induce apoptosis in CD4 + T-cells. In contrast, in our experiments, NSPC IL-10 or culture supernatants of NSPC IL-10 did not induce apoptosis of antigen-specific or polyclonal stimulation of spleen cells. We further investigated if IDO expression could be responsible for the inhibitory effects of NSPC IL-10 . IDO is the first and rate-limiting enzyme in the degradation of tryptophan to kynurenine [ 41 ]. In healthy individuals, IDO is expressed only at low levels but expression increases after infection or inflammation [ 25 ]. It was shown that in proteolipid protein (PLP)-induced EAE, IDO induction leads to reduced neuroinflammation [ 42 ] and that mesenchymal stem cells suppress proliferation of T-cells via expression of IDO [ 43 ]. NSPC IL-10 and NSPCs express IDO in culture as demonstrated by RT-PCR, but there was no association between IDO expression and inhibition of proliferation mediated by NSPC IL-10 .
IL-10 is an anti-inflammatory cytokine mainly produced by Th2 cells, and inhibits the activity of Th1 lymphocytes, natural killer (NK) cells and macrophages [ 16 ]. The inhibitory effects of IL-10 may be mediated by inhibition of the co-stimulatory signals CD80 and CD86 on APCs [ 44 ], or by induction of immunosuppressive regulatory T-cells (Tregs), such as Tr1, which in turn inhibit cell proliferation through production of IL-10 or transforming growth factor (TGF)-β [ 45 ]. In addition, it has previously been shown that stimulation of CD4 + T-cells in the presence of IL-10 induces a state of unresponsiveness with reduced proliferation and IL-2 production [ 46 ]. Our co-cultivation experiments using NSPC IL-10 showed that there was no decreased expression of CD80 or CD86 on antigen-specific stimulated spleen cells or a higher percentage of CD4 + FoxP3 + cells (data not shown), but we found reduced expression of IL2Rα, reduced IL-2 production and proliferation when spleen cells were stimulated in the presence of NSPC IL-10 . Therefore, IL-10 producing NSPCs seem to mediate their effects by inducing a non-responsive state in T-cells. In a recent study, Yang et al . [ 13 ] evaluated the effects of IL-10 producing neural stem cells on EAE. After intravenous injection, cells were detected in peripheral lymphoid organs and were shown to act via an immunosuppressive mechanism which was enhanced through IL-10 production. In contrast to our study, these experiments also showed a positive effect when cells were injected after onset of clinical EAE.
As proof of concept, we have shown that IL-10 producing NSPCs ameliorate the clinical disease course of EAE. NSPCs may be used as vehicles to transport immunomodulatory molecules to sites of T-cell activation and inflammation in order to attenuate autoimmune processes. These results, however, do not rule out immunomodulatory functions of NSPC IL-10 within the brain and further work is needed to explore this. The finding that NSPC IL-10 only marginally suppresses EAE disease severity when administered after EAE symptoms has been established and indicates that the time of administration of NSPC IL-10 critically determines its potency to suppress CNS autoimmunity. Whether such an approach would be feasible in patients with MS remains to be established.
Abbreviations
1-MT: 1-methyl-DL-tryptophan; AEC: 3-amino-9-ethylcarbazole; ANOVA: Analysis of variance; APC: Antigen-presenting cell; CFA: Complete Freund’s adjuvant; CNS: Central nervous system; ConA: Concanavalin A; DAPI: 4’,6-diamidino-2-phenylindole; EAE: Experimental autoimmune encephalomyelitis; EGF: Epidermal growth factor; ELISA: Enzyme-linked immunosorbent assay; ELISPOT: Enzyme-linked immunosorbent spot; FBS: Fetal bovine serum; FGF: Fibroblast growth factor; H&E: Hematoxylin and eosin; IDO: Indoleamine 2,3-dioxygenase; IFA: Incomplete Freund’s adjuvant; IFN: Interferon; IL: Interleukin; MHC: Major histocompatibility complex; MOG: Myelin oligodendrocyte glycoprotein; MOG35-55: Myelin oligodendrocyte glycoprotein aa 35–55; MS: Multiple sclerosis; MSCV: Murine stem cell virus; NK: Natural killer; NSPC: Neural stem/progenitor cell; PBS: Phosphate-buffered saline; PFA: Paraformaldehyde; PI: Propidium iodide; PLP: Proteolipid protein; PSA-NCAM: Polysialic acid neural cell adhesion molecule; RPMI: Roswell Park Memorial Institute; RT-PCR: Reverse transcription polymerase chain reaction; TCR: T-cell receptor; TGF: Transforming growth factor; Th: T-helper; TMB: 3,3’5,5’-tetramethylbenzidine; TNF: Tumor necrosis factor; Treg: Regulatory T-cell.
Competing interests
The authors declare that they have no competing interests.
Authors’ contributions
JK, BG and AM designed the experiments. JK performed experiments, JK and BG analyzed the data and performed statistical analysis. JK, BG and FB interpreted the data, wrote the manuscript and prepared the graphics. NOS, MD and JM provided critical materials. All authors read and approved the final version of the manuscript.
Contributor Information
Juliane Klose, Email: [email protected].
Nils Ole Schmidt, Email: [email protected].
Arthur Melms, Email: [email protected].
Makoto Dohi, Email: [email protected].
Jun-ichi Miyazaki, Email: [email protected].
Felix Bischof, Email: [email protected].
Bernhard Greve, Email: [email protected].
Acknowledgment
This research was supported by an Interdisciplinary Center for Clinical Research (IZKF) junior research group grant (1685-0-0) from the medical faculty of the University of Tübingen (Tübingen, Germany) to BG. We thank Nathalie Vetter, Markus Messing and Nada Hamdi for technical support.
- Frohman EM, Racke MK, Raine CS. Multiple sclerosis–the plaque and its pathogenesis. N Engl J Med. 2006;354:942–955. doi: 10.1056/NEJMra052130. [ DOI ] [ PubMed ] [ Google Scholar ]
- Rejdak K, Jackson S, Giovannoni G. Multiple sclerosis: a practical overview for clinicians. Br Med Bull. 2010;95:79–104. doi: 10.1093/bmb/ldq017. [ DOI ] [ PubMed ] [ Google Scholar ]
- Deierborg T, Roybon L, Inacio AR, Pesic J, Brundin P. Brain injury activates microglia that induce neural stem cell proliferation ex vivo and promote differentiation of neurosphere-derived cells into neurons and oligodendrocytes. Neuroscience. 2010;171:1386–1396. doi: 10.1016/j.neuroscience.2010.09.045. [ DOI ] [ PubMed ] [ Google Scholar ]
- Picard-Riera N, Decker L, Delarasse C, Goude K, Nait-Oumesmar B, Liblau R, Pham-Dinh D, Evercooren AB. Experimental autoimmune encephalomyelitis mobilizes neural progenitors from the subventricular zone to undergo oligodendrogenesis in adult mice. Proc Natl Acad Sci USA. 2002;99:13211–13216. doi: 10.1073/pnas.192314199. [ DOI ] [ PMC free article ] [ PubMed ] [ Google Scholar ]
- Reynolds BA, Tetzlaff W, Weiss S. A multipotent EGF-responsive striatal embryonic progenitor cell produces neurons and astrocytes. J Neurosci. 1992;12:4565–4574. doi: 10.1523/JNEUROSCI.12-11-04565.1992. [ DOI ] [ PMC free article ] [ PubMed ] [ Google Scholar ]
- Müller FJ, Snyder EY, Loring JF. Gene therapy: can neural stem cells deliver? Nat Rev Neurosci. 2006;7:75–84. doi: 10.1038/nrn1829. [ DOI ] [ PubMed ] [ Google Scholar ]
- Einstein O, Fainstein N, Vaknin I, Mizrachi-Kol R, Reihartz E, Grigoriadis N, Lavon I, Baniyash M, Lassmann H, Ben-Hur T. Neural precursors attenuate autoimmune encephalomyelitis by peripheral immunosuppression. Ann Neurol. 2007;61:209–218. doi: 10.1002/ana.21033. [ DOI ] [ PubMed ] [ Google Scholar ]
- Pluchino S, Zanotti L, Rossi B, Brambilla E, Ottoboni L, Salani G, Martinello M, Cattalini A, Bergami A, Furlan R, Comi G, Constantin G, Martino G. Neurosphere-derived multipotent precursors promote neuroprotection by an immunomodulatory mechanism. Nature. 2005;436:266–271. doi: 10.1038/nature03889. [ DOI ] [ PubMed ] [ Google Scholar ]
- Martino G, Pluchino S. The therapeutic potential of neural stem cells. Nat Rev Neurosci. 2006;7:395–406. doi: 10.1038/nrn1908. [ DOI ] [ PubMed ] [ Google Scholar ]
- Aboody KS, Brown A, Rainov NG, Bower KA, Liu S, Yang W, Small JE, Herrlinger U, Ourednik V, Black PM, Breakefield XO, Snyder EY. Neural stem cells display extensive tropism for pathology in adult brain: evidence from intracranial gliomas. Proc Natl Acad Sci USA. 2000;97:12846–12851. doi: 10.1073/pnas.97.23.12846. [ DOI ] [ PMC free article ] [ PubMed ] [ Google Scholar ]
- Sun L, Lee J, Fine HA. Neuronally expressed stem cell factor induces neural stem cell migration to areas of brain injury. J Clin Invest. 2004;113:1364–1374. doi: 10.1172/JCI20001. [ DOI ] [ PMC free article ] [ PubMed ] [ Google Scholar ]
- Chu K, Kim M, Jeong SW, Kim SU, Yoon BW. Human neural stem cells can migrate, differentiate, and integrate after intravenous transplantation in adult rats with transient forebrain ischemia. Neurosci Lett. 2003;343:129–133. doi: 10.1016/S0304-3940(03)00174-5. [ DOI ] [ PubMed ] [ Google Scholar ]
- Yang J, Jiang Z, Fitzgerald DC, Ma C, Yu S, Li H, Zhao Z, Li Y, Ciric B, Curtis M. et al. Adult neural stem cells expressing IL-10 confer potent immunomodulation and remyelination in experimental autoimmune encephalitis. J Clin Invest. 2009;119:3678–3691. doi: 10.1172/JCI37914. [ DOI ] [ PMC free article ] [ PubMed ] [ Google Scholar ]
- Asadullah K, Sterry W, Volk HD. Interleukin-10 therapy–review of a new approach. Pharmacol Rev. 2003;55:241–269. doi: 10.1124/pr.55.2.4. [ DOI ] [ PubMed ] [ Google Scholar ]
- Ding L, Linsley PS, Huang LY, Germain RN, Shevach EM. IL-10 inhibits macrophage costimulatory activity by selectively inhibiting the up-regulation of B7 expression. J Immunol. 1993;151:1224–1234. [ PubMed ] [ Google Scholar ]
- Moore KW, De-Waal MR, Coffman RL, O’Garra A. Interleukin-10 and the interleukin-10 receptor. Annu Rev Immunol. 2001;19:683–765. doi: 10.1146/annurev.immunol.19.1.683. [ DOI ] [ PubMed ] [ Google Scholar ]
- Bettelli E, Das MP, Howard ED, Weiner HL, Sobel RA, Kuchroo VK. IL-10 is critical in the regulation of autoimmune encephalomyelitis as demonstrated by studies of IL-10- and IL-4-deficient and transgenic mice. J Immunol. 1998;161:3299–3306. [ PubMed ] [ Google Scholar ]
- Xiao BG, Bai XF, Zhang GX, Link H. Suppression of acute and protracted-relapsing experimental allergic encephalomyelitis by nasal administration of low-dose IL-10 in rats. J Neuroimmunol. 1998;84:230–237. doi: 10.1016/S0165-5728(97)00264-6. [ DOI ] [ PubMed ] [ Google Scholar ]
- Hansen K, Müller FJ, Messing M, Zeigler F, Loring JF, Lamszus K, Westphal M, Schmidt NO. A 3-dimensional extracellular matrix as a delivery system for the transplantation of glioma-targeting neural stem/progenitor cells. Neuro Oncol. 2010;12:645–654. doi: 10.1093/neuonc/noq002. [ DOI ] [ PMC free article ] [ PubMed ] [ Google Scholar ]
- Bettelli E, Pagany M, Weiner HL, Linington C, Sobel RA, Kuchroo VK. Myelin oligodendrocyte glycoprotein-specific T cell receptor transgenic mice develop spontaneous autoimmune optic neuritis. J Exp Med. 2003;197:1073–1081. doi: 10.1084/jem.20021603. [ DOI ] [ PMC free article ] [ PubMed ] [ Google Scholar ]
- Greve B, Weissert R, Hamdi N, Bettelli E, Sobel RA, Coyle A, Kuchroo VK, Rajewsky K, Schmidt-Supprian M. I kappa B kinase 2/beta deficiency controls expansion of autoreactive T cells and suppresses experimental autoimmune encephalomyelitis. J Immunol. 2007;179:179–185. doi: 10.4049/jimmunol.179.1.179. [ DOI ] [ PubMed ] [ Google Scholar ]
- Sheng H, Wang Y, Jin Y, Zhang Q, Zhang Y, Wang L, Shen B, Yin S, Liu W, Cui L, Li N. A critical role of IFNgamma in priming MSC-mediated suppression of T cell proliferation through up-regulation of B7-H1. Cell Res. 2008;18:846–857. doi: 10.1038/cr.2008.80. [ DOI ] [ PubMed ] [ Google Scholar ]
- Galli R, Gritti A, Bonfanti L, Vescovi AL. Neural stem cells: an overview. Circ Res. 2003;92:598–608. doi: 10.1161/01.RES.0000065580.02404.F4. [ DOI ] [ PubMed ] [ Google Scholar ]
- Bischof F, Hofmann M, Schumacher TN, Vyth-Dreese FA, Weissert R, Schild H, Kruisbeek AM, Melms A. Analysis of autoreactive CD4 T cells in experimental autoimmune encephalomyelitis after primary and secondary challenge using MHC class II tetramers. J Immunol. 2004;172:2878–2884. doi: 10.4049/jimmunol.172.5.2878. [ DOI ] [ PubMed ] [ Google Scholar ]
- Mellor AL, Munn DH. IDO expression by dendritic cells: tolerance and tryptophan catabolism. Nat Rev Immunol. 2004;4:762–774. doi: 10.1038/nri1457. [ DOI ] [ PubMed ] [ Google Scholar ]
- Mellor AL, Munn DH. Tryptophan catabolism and T-cell tolerance: immunosuppression by starvation? Immunol Today. 1999;20:469–473. doi: 10.1016/S0167-5699(99)01520-0. [ DOI ] [ PubMed ] [ Google Scholar ]
- Einstein O, Karussis D, Grigoriadis N, Mizrachi-Kol R, Reinhartz E, Abramsky O, Ben-Hur T. Intraventricular transplantation of neural precursor cell spheres attenuates acute experimental allergic encephalomyelitis. Mol Cell Neurosci. 2003;24:1074–1082. doi: 10.1016/j.mcn.2003.08.009. [ DOI ] [ PubMed ] [ Google Scholar ]
- Parr AM, Tator CH, Keating A. Bone marrow-derived mesenchymal stromal cells for the repair of central nervous system injury. Bone Marrow Transplant. 2007;40:609–619. doi: 10.1038/sj.bmt.1705757. [ DOI ] [ PubMed ] [ Google Scholar ]
- Van-Wijmeersch B, Sprangers B, Rutgeerts O, Lenaerts C, Landuyt W, Waer M, Billiau AD, Dubois B. Allogeneic bone marrow transplantation in models of experimental autoimmune encephalomyelitis: evidence for a graft-versus-autoimmunity effect. Biol Blood Marrow Transplant. 2007;13:627–637. doi: 10.1016/j.bbmt.2007.03.001. [ DOI ] [ PubMed ] [ Google Scholar ]
- Kassis I, Grigoriadis N, Gowda-Kurkalli B, Mizrachi-Kol R, Ben-Hur T, Slavin S, Abramsky O, Karussis D. Neuroprotection and immunomodulation with mesenchymal stem cells in chronic experimental autoimmune encephalomyelitis. Arch Neurol. 2008;65:753–761. doi: 10.1001/archneur.65.6.753. [ DOI ] [ PubMed ] [ Google Scholar ]
- Li Y, Chen J, Chen XG, Wang L, Gautam SC, Xu YX, Katakowski M, Zhang LJ, Lu M, Janakiraman N, Chopp M. Human marrow stromal cell therapy for stroke in rat: neurotrophins and functional recovery. Neurology. 2002;59:514–523. doi: 10.1212/WNL.59.4.514. [ DOI ] [ PubMed ] [ Google Scholar ]
- Park HJ, Lee PH, Bang OY, Lee G, Ahn YH. Mesenchymal stem cells therapy exerts neuroprotection in a progressive animal model of Parkinson’s disease. J Neurochem. 2008;107:141–151. doi: 10.1111/j.1471-4159.2008.05589.x. [ DOI ] [ PubMed ] [ Google Scholar ]
- Ben-Hur T, Ben-Menachem O, Furer V, Einstein O, Mizrachi-Kol R, Grigoriadis N. Effects of proinflammatory cytokines on the growth, fate, and motility of multipotential neural precursor cells. Mol Cell Neurosci. 2003;24:623–631. doi: 10.1016/S1044-7431(03)00218-5. [ DOI ] [ PubMed ] [ Google Scholar ]
- Pluchino S, Quattrini A, Brambilla E, Gritti A, Salani G, Dina G, Galli R, Del-Carro U, Amadio S, Bergami A, Furlan R, Comi G, Vescovi AL, Martino G. Injection of adult neurospheres induces recovery in a chronic model of multiple sclerosis. Nature. 2003;422:688–694. doi: 10.1038/nature01552. [ DOI ] [ PubMed ] [ Google Scholar ]
- Schmidt NO, Przylecki W, Yang W, Ziu M, Teng Y, Kim SU, Black PM, Aboody KS, Carroll RS. Brain tumor tropism of transplanted human neural stem cells is induced by vascular endothelial growth factor. Neoplasia. 2005;7:623–629. doi: 10.1593/neo.04781. [ DOI ] [ PMC free article ] [ PubMed ] [ Google Scholar ]
- Schmidt NO, Koeder D, Messing M, Mueller FJ, Aboody KS, Kim SU, Black PM, Carroll RS, Westphal M, Lamszus K. Vascular endothelial growth factor-stimulated cerebral microvascular endothelial cells mediate the recruitment of neural stem cells to the neurovascular niche. Brain Res. 2009;1268:24–37. doi: 10.1016/j.brainres.2009.02.065. [ DOI ] [ PubMed ] [ Google Scholar ]
- Seabrook TJ, Littlewood-Evans A, Brinkmann V, Pöllinger B, Schnell C, Hiestand PC. Angiogenesis is present in experimental autoimmune encephalomyelitis and pro-angiogenic factors are increased in multiple sclerosis lesions. J Neuroinflammation. 2010;7:95. doi: 10.1186/1742-2094-7-95. [ DOI ] [ PMC free article ] [ PubMed ] [ Google Scholar ]
- Pluchino S, Gritti A, Blezer E, Amadio S, Brambilla E, Borsellino G, Cossetti C, Del-Carro U, Comi G, t Hart B, Vescovi A, Martino G. Human neural stem cells ameliorate autoimmune encephalomyelitis in non-human primates. Ann Neurol. 2009;66:343–354. doi: 10.1002/ana.21745. [ DOI ] [ PubMed ] [ Google Scholar ]
- Flugel A, Berkowicz T, Ritter T, Labeur M, Jenne DE, Li Z, Ellwart JW, Willem M, Lassmann H, Wekerle H. Migratory activity and functional changes of green fluorescent effector cells before and during experimental autoimmune encephalomyelitis. Immunity. 2001;14:547–560. doi: 10.1016/S1074-7613(01)00143-1. [ DOI ] [ PubMed ] [ Google Scholar ]
- Plumas J, Chaperot L, Richard MJ, Molens JP, Bensa JC, Favrot MC. Mesenchymal stem cells induce apoptosis of activated T cells. Leukemia. 2005;19:1597–1604. doi: 10.1038/sj.leu.2403871. [ DOI ] [ PubMed ] [ Google Scholar ]
- Takikawa O, Yoshida R, Kido R, Hayaishi O. Tryptophan degradation in mice initiated by indoleamine 2,3-dioxygenase. J Biol Chem. 1986;261:3648–3653. [ PubMed ] [ Google Scholar ]
- Kwidzinski E, Bunse J, Aktas O, Richter D, Mutlu L, Zipp F, Nitsch R, Bechmann I. Indolamine 2,3-dioxygenase is expressed in the CNS and down-regulates autoimmune inflammation. FASEB J. 2005;19:1347–1349. doi: 10.1096/fj.04-3228fje. [ DOI ] [ PubMed ] [ Google Scholar ]
- Shi Y, Hu G, Su J, Li W, Chen Q, Shou P, Xu C, Chen X, Huang Y, Zhu Z, Huang X, Han X, Xie N, Ren G. Mesenchymal stem cells: a new strategy for immunosuppression and tissue repair. Cell Res. 2010;20:510–518. doi: 10.1038/cr.2010.44. [ DOI ] [ PubMed ] [ Google Scholar ]
- De-Waal MR, Haanen J, Spits H, Roncarolo MG, Te-Velde A, Figdor C, Johnson K, Kastelein R, Yssel H, De-Vries JE. Interleukin 10 (IL-10) and viral IL-10 strongly reduce antigen-specific human T cell proliferation by diminishing the antigen-presenting capacity of monocytes via downregulation of class II major histocompatibility complex expression. J Exp Med. 1991;174:915–924. doi: 10.1084/jem.174.4.915. [ DOI ] [ PMC free article ] [ PubMed ] [ Google Scholar ]
- Vignali DA, Collison LW, Workman CJ. How regulatory T cells work. Nat Rev Immunol. 2008;8:523–532. doi: 10.1038/nri2343. [ DOI ] [ PMC free article ] [ PubMed ] [ Google Scholar ]
- Groux H, Bigler M, De-Vries JE, Roncarolo MG. Inhibitory and stimulatory effects of IL-10 on human CD8+ T cells. J Immunol. 1998;160:3188–3193. [ PubMed ] [ Google Scholar ]
- View on publisher site
- PDF (1.1 MB)
- Collections
Similar articles
Cited by other articles, links to ncbi databases.
- Download .nbib .nbib
- Format: AMA APA MLA NLM
Add to Collections
- Search Menu
- Sign in through your institution
- Advance articles
- Editor's Choice
- Review Articles
- Outstanding Merit Award
- Special Issues
- Why Publish in International Immunology?
- Author Guidelines
- Submission Site
- Open Access
- About International Immunology
- About the Japanese Society for Immunology
- Editorial Board
- For Reviewers
- Advertising and Corporate Services
- Journals Career Network
- Self-Archiving Policy
- Dispatch Dates
- Journals on Oxford Academic
- Books on Oxford Academic

Article Contents
Introduction, acknowledgements, author contributions, data availability.
- < Previous
Prevention of experimental autoimmune encephalomyelitis by targeting 6-sulfo sialyl Lewis X glycans involved in lymphocyte homing

- Article contents
- Figures & tables
- Supplementary Data
Qianqian Liu, Wei Xiong, Sachiyo Obara, Hirohito Abo, Hiroko Nakatsukasa, Hiroto Kawashima, Prevention of experimental autoimmune encephalomyelitis by targeting 6-sulfo sialyl Lewis X glycans involved in lymphocyte homing, International Immunology , Volume 36, Issue 6, June 2024, Pages 303–316, https://doi.org/10.1093/intimm/dxae009
- Permissions Icon Permissions
Lymphocyte homing to peripheral lymph nodes (PLN) is critical for immune surveillance. However, autoimmune diseases such as multiple sclerosis (MS) can occur due to excessive immune responses in the PLN. Here we show that 6-sulfo sialyl Lewis X (6-sulfo sLe x ) glycans on high endothelial venules that function as ligands for l -selectin on lymphocytes play a critical role in the pathogenesis of experimental autoimmune encephalomyelitis (EAE), an animal model of MS. In N -acetylglucosamine-6- O -sulfotransferase (GlcNAc6ST)-1 and GlcNAc6ST-2 double-knockout mice lacking the expression of 6-sulfo sLe X glycans, the EAE symptoms and the numbers of effector Th1 and Th17 cells in the draining lymph nodes (dLN) and spinal cords (SC) were significantly reduced. To determine whether 6-sulfo sLe X could serve as a target for MS, we also examined the effects of anti-glycan monoclonal antibody (mAb) SF1 against 6-sulfo sLe X in EAE. Administration of mAb SF1 significantly reduced EAE symptoms and the numbers of antigen-specific effector T cells in the dLN and SC in association with suppression of critical genes including Il17a and Il17f that are involved in the pathogenesis of EAE. Taken together, these results suggest that 6-sulfo sLe X glycan would serve as a novel target for MS.

Multiple sclerosis (MS) is an autoimmune disease resulting from the immune system’s erroneous targeting of myelin proteins in the central nervous system (CNS), including the brain and spinal cord. This immune disorder leads to demyelination and hampers neural signal transmission, resulting in functional impairments ( 1 ). Current MS treatments include the use of steroids, interferon-β (IFN-β), glatiramer acetate, fumaric acid derivatives, sphingosine-1-phosphate receptor modulators, Bruton’s tyrosine kinase inhibitors, and B-cell-depleting antibodies ( 2–4 ). These agents improve the patient condition by suppressing inflammatory reactions or regulating lymphocyte migration. However, these therapeutic agents are associated with a range of unavoidable side effects, including flu-like symptoms, cardiac arrhythmias, and life-threatening brain infections. Furthermore, a definitive cure for MS has yet to be developed. Hence, we consider the augmentation of therapeutic drug options highly valuable.
Experimental autoimmune encephalomyelitis (EAE), a widely used animal model that mimics MS, has been extensively used in basic scientific and preclinical studies ( 5 ). In this model, immunization with myelin oligodendrocyte glycoprotein (MOG) peptide induces the migration of activated T lymphocytes into the CNS, which is a critical step in disease onset ( 6 ). Different subsets of effector T cells play distinct roles in EAE development. For instance, the CD4 + T-cell subsets Th1 and Th17, identified by their respective expression of T-bet and RORγt (essential transcription factors for Th1 and Th17 differentiation, respectively), facilitate the development of EAE ( 7 , 8 ). Memory-like CD4 + T cells (CD25 − CD62L low CD44 high ) predominantly produce IL-17A and IFN-γ following cytokine stimulation and infiltrate into the CNS, thereby driving EAE progression ( 9 ). In addition to CD4 + T cells, IFN-γ-producing CD8 + T cells also significantly contribute to EAE induction ( 10 , 11 ). Considering the increased presence of central and effector memory phenotypes of CD8 + T cells in the cerebrospinal fluid of MS patients, these cells are considered to be associated with MS pathogenesis ( 12 , 13 ). Therefore, T-cell differentiation and transmigration into the CNS are considered as targets for MS treatment.
After undergoing a series of developmental processes within the primary lymphoid organs, including the bone marrow and thymus, lymphocytes are transported to peripheral lymphoid organs throughout the body via blood vessels ( 14 ). This unique migration process, known as lymphocyte homing, enables lymphocytes to perform their functions in various tissues, organs, and sites of inflammation ( 15 ). High endothelial venules (HEV), a specialized blood vessel type, are present in peripheral lymphoid tissues ( 14 ). During initiation of lymphocyte homing to peripheral lymph nodes (PLN), naïve lymphocytes interact with 6-sulfo sialyl Lewis X (6-sulfo sLe x ) on the surface of HEV through their lymphocyte homing receptor l -selectin ( 16 ), where they differentiate into different subtypes such as Th1, Th17, and IFN-γ + CD8 + effector T cells and are induced to proliferate under the stimulation of cognate antigens, chemokines, and cytokines ( 17 ). A glycan 6-sulfo sLe x , sialyl-α(2-3)-galactopyranosyl-β(1-4)-[fucopyranosyl-α(1-3)(sulfo-6)]- N -acetylglucosamine, is highly expressed on the surface of HEV in PLN, and is capable of binding to l -selectin. N -acetylglucosamine-6- O -sulfotransferase (GlcNAc6ST)-1 and GlcNAc6ST-2 are two GlcNAc-6- O -sulfotransferases essential for 6-sulfo sLe X biosynthesis ( 18 ). GlcNAc6ST-1 and -2 double knockout (DKO) mice reportedly display approximately 75% less lymphocyte homing to the PLN than wild-type (WT) mice do ( 19 , 20 ). Despite the biological importance of 6-sulfo sLe X and naïve-lymphocyte homing in homeostasis, their significance in EAE remains unexplored.
We recently developed the novel monoclonal antibody (mAb) SF1, which specifically binds to 6-sulfo sLe X by recognizing the sulfate group and the fucose and sialic acid residues ( 21 ). As indicated in that study, SF1 specifically binds to HEV in the PLN. This binding disrupts the interaction between l -selectin and HEV, effectively impeding lymphocyte homing to the PLN and nasal-associated lymphoid tissue. Therefore, mAb SF1 could be a potential therapeutic agent against various immune-related diseases by mitigating immune responses through disruption of lymphocyte trafficking.
In this study, we evaluated the role of 6-sulfo sLe X in an EAE model using GlcNAc6ST-1/2 double-deficient mice and the SF1 antibody. We initially demonstrated that the severity of EAE in DKO mice was significantly reduced compared to that in WT mice, with a remarkable reduction in effector T cells in the spinal cord and draining lymph nodes (dLN). In addition, SF1 administration, which suppresses lymphocyte homing, greatly reduced the severity of EAE and effector T-cell numbers in dLN. This treatment significantly reduced the expression of chemokines and inflammatory cytokines in CD4 + T cells, leading to suppression of EAE pathogenesis. Collectively, our data demonstrate that 6-sulfo sLe X highly contributes to EAE and that inhibition of the interaction between l -selectin and 6-sulfo sLe X by SF1 efficiently ameliorates EAE pathogenesis. Our findings suggest a novel immune therapeutic approach for the treatment of MS.
Female C57BL/6 and ICR nu/nu mice were purchased from Charles River Laboratories (Yokohama, Japan). GlcNAc6ST-1/-2 DKO mice were maintained as previously described ( 20 , 21 ). The permission to use these mice in this study was obtained from the original inventor, Dr Minoru Fukuda (Sanford Burnham Prebys Medical Discovery Institute). Mice were acclimated to a housing system (LP-30LED-8CTAR, NK system) at 25°C for 1 week. Mice at the age of 8 weeks were used for the experiments and treated according to the guidelines of the Institutional Animal Care and Use Committees of Chiba University. The reporting in the manuscript follows the recommendations in the ARRIVE (Animals in Research: Reporting In Vivo Experiments) guidelines.
Antibody preparation
The SF1 antibody was prepared as previously described ( 21 ). Briefly, pristane (0.1 ml/mouse) was injected intraperitoneally using a 26-gauge needle and an all-plastic syringe. Hybridoma cells that secreted SF1 were cultured and intraperitoneally injected into ICR nu/nu mice (5 × 10 6 cells/mouse) 2 weeks after pristane injection. After 1–2 weeks, the ascitic fluid was harvested. SF1 was purified using the caprylic acid (FUJIFILM Wako, Osaka, Japan) precipitation method for impurity reduction and the ammonium sulfate method for precipitation.
Experimental autoimmune encephalomyelitis
To induce EAE, C57BL/6 mice, or GlcNAc6ST-1/-2 DKO mice were subcutaneously injected with 0.1 mg of MOG 35–55 peptide (JH, Japan) emulsified in complete Freund’s adjuvant (CFA, Difco, USA) containing Mycobacterium tuberculosis H37Ra (1 mg/ml) (Difco, USA) at two different sites on the hind flank on day 0, followed by intraperitoneal injection of 200 ng pertussis toxin (PTX, CosmoBio, Japan). On day 2, mice received an additional intraperitoneal injection of 200 ng of PTX. In some experiments, mice were intraperitoneally injected with 0.2 mg SF1 antibody or 0.2 ml PBS 3 h before PTX injection on days 0 and 2. Clinical scores were evaluated daily, according to the following criteria: 0 normal, 1 low tail tonus, 2 tail completely dropped, 3 abnormal motility, 4 complete hind limb weakness, 5 forelimb paralysis, 6 death. On days 5 and 12, mice were euthanized for analyses.
Lymphocyte preparation
Lymphocytes from inguinal lymph nodes (iLN) were re-suspended by mechanical disruption and passed through a 70-μm mesh. Approximately 3.5 cm of the mouse spinal cord was collected from the tail end to the head. Thereafter, the central 0.5 cm was used for sectioning, while the remaining portion was subjected to digestion. Spinal cords were digested with 1 mg/ml collagenase P (Roche, USA), 0.2 mg/ml dispase I (Gibco, USA), and 0.01 mg/ml DNase I (Roche) in RPMI-1640 medium containing 5% fetal bovine serum (FBS) at 37°C with gentle agitation for 1 h. Lymphocytes in the spinal cord were suspended in 37% percoll and laid onto 70% percoll, followed by centrifugation at 2000 rpm for 20 min at 20°C, and re-suspended in RPMI-1640 medium for fluorescence-activated cell sorting (FACS) analysis and RNA sequencing.
Flow cytometry
Cellular staining was performed using the following antibodies: anti-CD3ε-BV421 (clone 145-2C11, BioLegend, USA, dilution 1:200), anti-CD4-APC-Cy7 (clone RM4-5, eBioscience, USA, dilution 1:200), anti-CD8α-PE (clone 53-6.3, BioLegend, dilution 1:200), anti-CD62L-APC (clone MEL-14, BioLegend, dilution 1:200), anti-CD44-FITC (clone IM7, BioLegend, dilution 1:200), anti-CD3ε-FITC (clone 145-2C11, BioLegend, dilution 1:200), anti-CD8α-PE-Cy7 (clone 53-6.7, BioLegend, dilution 1:200), anti-IFN-γ-BV421 (clone XMG1.2, BioLegend, dilution 1:200), and anti-IL-17A-PE (clone eBio17B7, eBioscience, dilution 1:200). The following antibodies were used for cell sorting: anti-CD45-APC-Cy7 (clone 30-F11, BioLegend, dilution 1:200), anti-CD3ε-APC (clone 145-2C11, BioLegend, dilution 1:200), anti-CD4-PE (clone RM4-5, BioLegend, dilution 1:200), anti-CD19-FITC (clone 1D3, BioLegend, dilution 1:200), anti-CD16/32 (clone 2.4G2, BioLegend, dilution 1:200), and anti-CD4-FITC (clone RM4-5, BioLegend, dilution 1:200). To detect surface markers, cells were incubated for 20 min at 4°C. For intracellular staining of cytokines, cells were stimulated with Brefeldin A and Cell Activation Cocktail (BioLegend) for 4 h at 37°C. After staining with Zombie Aqua dye (BioLegend) and surface markers, cells were fixed and permeabilized with Foxp3/Transcription Factor Staining Buffer Set (ThermoFisher, USA) for 20 min at 25°C. Cells were then incubated with intracellular markers for 20 min and subjected to FACS analysis. For cell sorting, cells were incubated with anti-CD16/32 for 10 min, then incubated with antibodies against cell surface markers for 20 min at 4°C. All samples were analyzed using a CytoFLEX flow cytometer (Beckman Coulter, USA), and the data were analyzed using FlowJo software.
Immunohistochemistry
Spinal cords were embedded in Tissue-Tek O.C.T. Compound (Sakura, Japan) and frozen in solid blocks ready for sectioning. For Luxol fast blue staining, frozen sections of the spinal cord were sliced at 14 μm thickness, and defatted in 80% ethanol for 3 h, followed by incubation in a 0.1% Luxol fast blue solution for 2 h at 60°C. After sections were cooled to room temperature, they were washed with 100% ethanol for 5 min, followed by washing in water for 5 min. Sections were then incubated in a 0.05% lithium carbonate solution for more than 15 min until the gray matter became colorless and the white matter remained blue. Sections were counterstained using a 0.1% cresyl violet solution for 5 min, washed in water, dehydrated in 80% and 100% ethanol, and rinsed in xylene. All images were obtained using an all-in-one fluorescence microscope (BZ-X800, Keyence), and the demyelinated area was analyzed using ImageJ software (v 1.53).
RNA sequencing
CD45 + 7-AAD − CD3ε + CD4 + T cells from the iLN of PBS- or SF1-treated C57BL/6 mice were sorted on day 5 post-EAE induction using a BD FACSAria III flow cytometer with a 70-μm nozzle and maintained in RPMI medium. The sorted cells were then centrifuged, and RNA was extracted using a Direct-zol RNA MiniPrep Kit (ZYR). Sequencing was performed using a DNBSEQ-G400 (MGI Tech). Further, transcriptome sequencing experiments, including RNA extraction and QC, library construction and purification, library QC and quantitation, sequencing cluster generation, and high-throughput sequencing, were performed. After mixing libraries on the basis of their effective concentrations and the required sequencing data volume, the DNBSEQ platform was used for high-throughput sequencing with alignment to a chosen reference (Mus musculus Ensembl GRCm38.101). Differential gene analysis was performed using the Bioconductor packages DESeq2 (V1.6.3) and edgeR (V3.4.6). Part of the data visualization was created using http://www.bioinformatics.com.cn , an online platform for data analysis and visualization. PCA was performed using the deep tool plot PCA in Galaxy. A volcano map was generated using the Volcano Plot in Galaxy.
Statistical analyses
Data were presented as the mean ± standard error of the mean (SEM), or mean ± standard deviation (SD). Statistical analyses were performed using GraphPad Prism 9. A normal distribution was assumed, and no formal tests were conducted. Depending on the data type, statistical significance was determined using an unpaired Student’s t -test, multiple unpaired t -tests, or one- or two-way analysis of variance (ANOVA). For sequencing data statistics, please refer to the relevant sections. P values less than or equal to .05 were considered significant (* P < .05, ** P < .01, *** P < .001).
GlcNAc6ST-1/2 double-deficiency ameliorates EAE symptoms and effector T-cell accumulation in the CNS
To explore the role of 6-sulfo sLe X in EAE, we induced EAE in WT and GlcNAc6ST-1 and 2 DKO mice ( Fig. 1A ). The onset of EAE in DKO mice was markedly delayed from day 7 to day 10, and the severity of the disease course was significantly reduced compared to that in WT mice ( Fig. 1B ). A longer-term experiment of clinical score and Kaplan–Meier plot indicated that this suppressive effect of WT/DKO mice was observed until day 28 ( Supplementary Fig. 1 ). On day 12, Luxol fast blue staining of spinal cord sections revealed that WT mice displayed significantly more demyelination compared with DKO mice, indicating less nerve damage in DKO mice ( Fig. 1C ). We next analyzed the immune cell subsets related to EAE pathogenesis in the spinal cord by flow cytometry on days 0, 5, and 12. On day 12, the number of total CD4 + T, Th1, Th17, and IFN-γ + CD8 + T cells in the spinal cord of DKO mice was significantly lower than those in WT mice, with no significant increase compared to those on day 0 ( Fig. 1E ), as determined by the total number of lymphocytes and the percentage of each cell population ( Fig. 1D ) in the spinal cord. To investigate whether deficiency of GlcNAc6ST-1 and 2 affects the memory T-cell population, we also analyzed CD4 + CD44 + CD62L − effector memory T (CD4 + Tem), CD4 + CD44 + CD62L + central memory T (CD4 + Tcm), CD8 + CD44 + CD62L − effector memory T (CD8 + Tem), and CD8 + CD44 + CD62L + central memory T (CD8 + Tcm) cells in the spinal cord using flow cytometry ( Fig. 1F , G ). The number of CD4 + Tem, CD4 + Tcm, CD8 + Tem, and CD8 + Tcm cells in the spinal cord of WT mice gradually increased, reaching the highest levels on day 12. In contrast, the number of these T-cell subsets in the spinal cords of DKO mice was significantly reduced compared to WT mice. These findings suggest that a deficiency in GlcNAc6ST-1/2 delays the onset and reduces severity in the EAE model, which is accompanied by decreasing the number of effector T cells into the CNS.

Suppression of EAE in GlcNAc6ST-1/2 double-deficient mice. (A) Schematic of the EAE induction protocol. EAE was induced in GlcNAc6ST-1 and -2 double knockout (DKO) mice and wild-type (WT) C57BL/6 mice. (B) Clinical EAE scores were monitored for 12 days ( n = 8). Mice were examined daily using a clinical EAE scoring system as described in the Methods section. Data are representative of six independent experiments. (C) Luxol Fast Blue staining of spinal cord sections on day 12 ( n = 3). Left, representative images of each group. Arrows indicate the demyelinated area. Scale bar, 200 μm. Right, quantification of the demyelinated area in the white matter. Data are representative of two independent experiments. (D) Representative FACS plots of Th1, Th17 (gated on CD3ε + CD4 + cells), and IFN-γ + CD8 + T cells (gated on CD3ε + CD8 + cells) in the spinal cord on day 12. Data are representative of three independent experiments. (E) Absolute cell numbers of total CD4 + T cells, Th1, Th17, and IFN-γ + CD8 + T cells in the spinal cord at different time points ( n = 4) (day 0, day 5, and day 12). Data are representative of three independent experiments. (F) Representative FACS plots of CD4 + effector memory T (Tem), central memory T (Tcm) (gated on CD3ε + CD4 + cells), CD8 + Tem, and CD8 + Tcm cells (gated on CD3ε + CD8 + cells) in the spinal cord on day 12. Data are representative of two independent experiments. (G) Absolute numbers of CD4 + Tem, CD4 + Tcm, CD8 + Tem, and CD8 + Tcm cells in the spinal cord of WT and DKO mice at different time points ( n = 4). Data are representative of two independent experiments. Statistical significance was assessed using a two-tailed unpaired t -test for comparisons between WT and DKO mice, and two-way ANOVA was utilized for comparisons among multiple groups over different time points. Levels of significance are denoted as follows: * P < .05, ** P < .01, *** P < .001.
GlcNAc6ST-1/2 double-deficiency suppresses T-cell numbers in the dLN
Drainage of antigen to the dLN is crucial for the initiation and development of EAE ( 22–24 ). Therefore, we investigated whether GlcNAc6ST-1/2 double-deficiency influences T-cell subpopulations in the iLN where the antigen drains into in our EAE model. For this, on day 5 after injection of the antigen peptide emulsified in CFA, we analyzed lymphocyte populations in the iLN of WT and DKO mice. Compared to WT mice, the number of CD4 + CD44 − CD62L + naive T, CD4 + Tem, CD4 + Tcm, CD8 + CD44 − CD62L + naive T, and CD8 + Tem cells in DKO mice was significantly decreased ( Fig. 2B ), as determined by the total number of lymphocytes and the percentage of each cell population ( Fig. 2A ) in the dLN. Moreover, total CD4 + T, CD4 + IFN-γ + (Th1), CD4 + IL-17 + (Th17), and IFN-γ + CD8 + T cells were significantly reduced in DKO mice compared to those in WT mice ( Fig. 2C , D ). These results suggest that deficiency of GlcNAc6ST-1/2 suppresses the increase of activated T-cell numbers in the dLN, which leads to significant suppression of the EAE symptoms described above.

6-Sulfo sLe X is required for effector T-cell accumulation in lymph nodes during EAE. (A) Representative FACS plots of CD4 + naive T, CD4 + Tem, CD4 + Tcm, CD8 + naive T, CD8 + Tem, and CD8 + Tcm cells in the iLN on day 5. Data are representative of two independent experiments. (B) Absolute cell numbers of CD4 + naive T, CD4 + Tem, CD4 + Tcm, CD8 + naive T, CD8 + Tem, and CD8 + Tcm cells in the iLN of WT and DKO mice on day 5 ( n = 4). Data are representative of two independent experiments. (C) Representative FACS plots of Th1 and Th17 cells in the iLN on day 5. Data are representative of five independent experiments. (D) Absolute numbers of total CD4 + T cells, Th1, Th17, and IFN-γ + CD8 + T cells in the iLN of WT and DKO mice on day 5 ( n = 4). Data are representative of five independent experiments. Statistical significance was assessed using two-tailed unpaired t -tests for comparisons between WT and DKO mice. Levels of significance are denoted as follows: * P < .05, ** P < .01, *** P < .001.
SF1 treatment ameliorates EAE symptoms and effector T-cell accumulation in CNS
Our group recently developed a mAb, SF1, that specifically binds to 6-sulfo sLe X on the surface of HEV by recognizing the sulfate group, fucose, and sialic acid residues, thus blocking the interaction between 6-sulfo sLe X and l -selectin on lymphocytes ( 21 ). On the bais of the present findings that the absence of 6-sulfo sLe X reduces the severity of EAE, we hypothesized that the SF1 antibody would ameliorate EAE severity. To investigate the effect of SF1 in this EAE model, we administered SF1 to WT mice by intraperitoneal injection on days 0 and 2 ( Fig. 3A ). Clinical scores indicated that the onset of disease in the SF1 group was significantly delayed, and the severity of the disease was notably reduced compared to that in the PBS control group ( Fig. 3B ). A longer-term experiment of clinical score and Kaplan–Meier plot indicated that this suppressive effect of PBS/SF1 mice was observed until day 28 ( Supplementary Fig. 2 ). On day 12, spinal cord sections were obtained from both groups, and Luxol fast blue staining was performed. Calculated demyelinated areas showed that the degree of demyelination in the SF1 group was significantly lower than that in the PBS group ( Fig. 3C ). Analyses of immune cell numbers in the spinal cord revealed that the number of total CD4 + T, Th1, Th17, and IFN-γ + CD8 + T cells was significantly lower in the SF1 group than in the PBS group ( Fig. 3E ), as determined by the total number of lymphocytes ( Supplementary Fig. 3 ) and the percentage of each cell population ( Fig. 3D ) in the spinal cord. The number of CD4 + Tcm, CD8 + Tem, and CD8 + Tcm cells in the spinal cord of the SF1 group was also significantly lower than that in the control group ( Fig. 3F , G ). We also performed an experiment using normal mouse IgG as a control instead of using PBS, and found significant suppressive activity of SF1 antibody compared to the control on the clinical score of EAE and accumulation of lymphocytes in the spinal cord ( Supplementary Fig. 4A, B ) without reducing the number of total lymphocytes in the spleen and blood ( Supplementary Fig. 4C, D ). In addition, we observed that, after SF1 injection into C57BL/6 WT mice, the number of total lymphocytes and their subpopulations (CD19 + B cells, CD3ε + T cells, CD4 + and CD8 + T cells) in the blood and spleen did not change significantly compared to the PBS group ( Supplementary Fig. 5 ), excluding the possibility that SF1 antibody is immunotoxic and systemically reduces the total number of T cells. Collectively, these findings suggest that the SF1 antibody ameliorates EAE by decreasing the numbers of Th1, Th17, and IFN-γ + CD8 + T cells in iLN, and consequently those in CNS.

Treatment with SF1 antibody ameliorates EAE. (A) Schematic of the EAE induction protocol. EAE was induced and SF1 antibody was administrated at day 0 and day 2. (B) Clinical EAE scores were monitored for 12 days (PBS, n = 5; SF1, n = 7). Mice were examined daily using a clinical EAE scoring system as described in the “Methods” section. Data are representative of four independent experiments. (C) Luxol Fast Blue staining of spinal cord sections on day 12 ( n = 3). Left, representative images of PBS and SF1 groups. Scale bar, 200 μm. Arrows indicate the demyelinated area. Right, quantification of the demyelinated area in the white matter. Data are representative of two independent experiments. (D) Representative FACS plots of Th1, Th17, and IFN-γ + CD8 + T cells in the spinal cord on day 12. Data are representative of three independent experiments. (E) Absolute cell numbers of total CD4 + T cells, Th1, Th17, and IFN-γ + CD8 + T cells in the spinal cord at different time points (day 0, day 5, and day 12) ( n = 4). Data are representative of three independent experiments. (F) Representative FACS plots of CD4 + effector memory T (Tem), central memory T (Tcm), CD8 + Tem, and CD8 + Tcm cells in the spinal cord on day 12. Data are representative of three independent experiments. (G) Absolute cell numbers of CD4 + Tem, Tcm, CD8 + Tem, and CD8 + Tcm cells in the spinal cord of PBS and SF1 groups at different time points ( n = 4). Data are representative of three independent experiments. Statistical significance was assessed using two-tailed unpaired t -tests for comparisons between PBS and SF1 groups, and two-way ANOVA was utilized for comparisons among multiple groups over different time points. Levels of significance are denoted as follows: * P < .05, ** P < .01, *** P < .001.
SF1 suppresses the numbers of antigen-specific T-cell subpopulations in the dLN
To explore the primary mechanism by which the SF1 antibody suppresses EAE symptoms, we further investigated the effects of mAb SF1 on T-cell subpopulations in the dLN during the early onset of EAE. On day 5 post-EAE induction, the number of CD4 + naive T, CD4 + Tem, CD4 + Tcm cells, CD8 + naïve T, CD8 + Tem, and CD8 + Tcm cells in the iLN in the SF1 group was significantly lower than those in the PBS group ( Fig. 4B ), as determined by the total number of lymphocytes and the percentage of each cell population ( Fig. 4A and Supplementary Fig. 6 ) in the dLN. Additionally, the administration of SF1 significantly reduced the number of total CD4 + T cells, Th1, Th17, and IFN-γ + CD8 + T cells ( Fig. 4C , D ). In addition, we found that the number of Treg cells in the spinal cord and dLN decreased in the SF1-administered mice ( Supplementary Fig. 7 ), suggesting that the preventive effects of SF1 is probably not due to the increase of Treg cells on day 5. MOG-specific CD4 + T cells are responsible for the development of EAE, and the expansion of myelin-specific T cells leads to severe EAE symptoms ( 25 ). Therefore, we analyzed MOG-specific CD4 + T cells using the antigen I-A b MOG 35–55 tetramer. We observed that the number of I-A b MOG 35–55 tetramer + MOG-specific CD4 + T cells in the iLN and spinal cord of the SF1 group was significantly lower than that in the PBS group ( Fig. 4E , F ), suggesting that the SF1 antibody decreased the number of antigen-specific T-cell subpopulations in the dLN in the EAE model.

SF1 suppresses activation of MOG-specific CD4 + T cells in the early onset of EAE. (A) Representative FACS plots of CD4 + naive T, CD4 + Tem, CD4 + Tcm, CD8 + naive T, CD8 + Tem, and CD8 + Tcm cells in the iLN on day 5. Data are representative of five independent experiments. (B) Absolute cell numbers of CD4 + naive T, CD4 + Tem, CD4 + Tcm, CD8 + naive T, CD8 + Tem, and CD8 + Tcm cells in the iLN of PBS and SF1 groups on day 5 ( n = 5). Data are representative of five independent experiments. (C) Representative FACS plots of Th1 and Th17 cells in the iLN on day 5. Data are representative of five independent experiments. (D) Absolute cell numbers of total CD4 + T cells, Th1, Th17, and IFN-γ + CD8 + T cells in the iLN of PBS and SF1 groups on day 5 ( n = 4). Data are representative of five independent experiments. (E) Representative FACS plots of CD4 + I-A b MOG 35–55 Tetramer + T cells in iLN; right, absolute numbers of CD4 + I-A b MOG 35–55 Tetramer T cells in iLN on day 5 ( n = 5). Data are representative of two independent experiments. (F) Representative FACS plots of CD4 + CD44 + I-A b MOG 35–55 Tetramer + T cells in the spinal cord on day 12 ( n = 5). Data are representative of two independent experiments. Statistical significance was assessed using two-tailed unpaired t -tests for comparisons between PBS and SF1 groups. Levels of significance are denoted as follows: * P < .05, ** P < .01, *** P < .001.
SF1 suppresses the expression of various genes involved in EAE pathogenesis
To explore the mode of SF1 action in more detail, we performed RNA sequencing of CD4 + T cells in the dLN of the EAE model. For this, CD4 + T cells in the iLN were sorted on day 5 after immunization with MOG peptides, and their total RNA was extracted for cDNA library construction. As shown in the heat map, we detected remarkable differences in the top 100 differentially expressed genes (DEGs) between the PBS and SF1 groups ( Fig. 5A ). Principal component analysis (PCA) also revealed that gene expression differences between PBS and SF1 groups showed distinct clusters, indicating that the administration of the SF1 antibody altered gene expression in CD4 + T cells ( Fig. 5B ). The overall distribution of DEGs is presented as a volcano plot, with 258 downregulated and 311 upregulated genes in the SF1 group compared to those in the PBS group ( Fig. 5C ). Inflammation- and lymphocyte migration-related genes were selected and labeled. The inhibition of cell homing could potentially lead to a decrease in transcription factor expression by altering cell activation status, cell responses to microenvironments, and signaling pathways ( 26 ). A previous study indicated that the STAT3 pathway is required for the development of EAE and influences naive T-cell differentiation into the Th17 phenotype ( 27 ). The results in Fig. 5C indicate that Stat3 was consistently downregulated in the SF1 group compared to its expression in the PBS group. Additionally, cytokines Il17a , Il17f , and Il22, and the cytokine receptor Ifngr1 , all of which exacerbate EAE severity ( 28 ), were downregulated by SF1 administration. Gene ontology (GO) analysis of the top 3000 DEGs involved in biological processes, cellular components, and molecular functions indicated that the PBS group was more active in cytokine-mediated signaling, immune receptor activation, cytokine binding, and cytokine receptor activity than the SF1 group ( Fig. 5D ). Additionally, the Gene Set Enrichment Analysis (GSEA) was also performed. The normalized enrichment score correlated with the GSEA plot results, showing that pathways related to the inflammatory response, IFN-γ response, IL2-STAT5 signaling, and PI3K-AKT-mTOR signaling were downregulated in the SF1 group ( Fig. 5E , F ). These results demonstrated that SF1 effectively inhibits the expression of cytokine-related genes and suppresses the immune response in the iLN of EAE models.

SF1 downregulates various genes involved in EAE pathogenesis in CD4 + T cells. CD45 + 7-AAD − CD3ε- + CD4 + T cells were sorted from iLN of PBS- or SF1-treated C57BL/6 mice on day 5 of EAE. (A) Heat map of the top 100 DEGs in PBS and SF1 groups (50 upregulated genes and 50 downregulated genes). Left, PBS group ( n = 3); right, SF1 group ( n = 3). (B) Principal component analysis (PCA) of CD4 + T cells in PBS and SF1 groups. (C) Volcano plot with 258 downregulated and 311 upregulated in the SF1 group compared to the PBS group. Cutoff values fold change >2 and P -value < .01 were utilized to identify differentially expressed genes. Unchanged genes are shown in gray. (D) Gene ontology (GO) analysis of the top 3000 differentially expressed genes, demonstrating biological processes (BP), cellular components (CC), and molecular functions (MF) enrichment in PBS vs. SF1. (E) Gene set enrichment analysis (GSEA) of CD4 + T cells from PBS and SF1 treated mice. Right bars indicate enriched gene sets in PBS group compared to those in SF1 group. Non-significant genes are shown in gray; significance, NOM P -value < .05. (F) GSEA plot showing gene enrichment signatures associated with inflammatory response, IFN-γ response, TGF-β signaling, TNFA signaling via NFκB, IL2-STAT5 signaling, and PI3K-AKT-mTOR signaling between PBS and SF1 CD4 + T cells; FDR, false-discovery rate.
In this study, we provide evidence that 6-sulfo sLe X glycan can serve as a novel target for the prevention of MS using sulfotransferase DKO mice and a recently developed anti-glycan mAb SF1 that is highly specific to this glycan with a strong blocking ability on l -selectin-mediated lymphocyte homing to PLN ( 21 ). Our results showed that the pathogenesis of EAE, a murine model of MS, was significantly suppressed in the DKO mice and in the SF1-treated WT mice in relation to the reduction of the total cell numbers of antigen-specific T cells in the dLN and CNS. The results of this study suggest that blocking of 6-sulfo sLe X glycan-mediated immune cell trafficking to PLN could prevent MS.
GlcNAc6ST-1 and 2 enzymes are responsible for the sulfation of 6-sulfo sLe X glycans. The binding of 6-sulfo sLe X to l -selectin plays a critical role in the adhesion and migration of lymphocytes to the PLN, as evidenced in studies using GlcNAc6ST-1 and 2 DKO mice ( 19 , 20 ). It was reported that GlcNAc6ST-1 knockout mice showed fewer clinical symptoms in EAE ( 29 ). However, it was not clear whether lymphocyte homing to PLN is involved in EAE because GlcNAc6ST-1 knockout mice showed both reduced expression of brain keratan sulfate and a 20% reduction in lymphocyte homing to PLN ( 30 ). Consistent with this report, our current study also showed the reduction of EAE in the GlcNAc6ST-1 and -2 DKO mice with approximately 75% less homing of lymphocytes to the PLN ( 20 ), although it was still uncertain whether lymphocyte homing is involved in the EAE onset because of the lack of GlcNAc6ST-1 involved in the brain keratan sulfate biosynthesis in these mutant mice. Therefore, we conducted further study using the SF1 antibody, which specifically binds to 6-sulfo sLe X glycans on HEV in PLN. Because 6-sulfo sLe X glycans recognized by mAb SF1 are specifically expressed in HEV in PLN but not in the CNS ( 21 ), it should be reasonable to consider that the sulfated glycan-mediated lymphocyte homing is indeed involved in EAE. Consistently, previous study using rapamycin-encapsulated microparticles indicated the importance of the PLN in the EAE pathological process ( 31 ). We also found significant suppression of Th17 cell numbers after SF1 antibody administration. Activation of naïve T cells depends on the recognition of antigens in the dLN ( 32 ). Therefore, our results suggest that without 6-sulfo sLe x , the efficiency with which naïve T cells encounter their cognate antigens presented by antigen-presenting cells in the lymph nodes is reduced, which potentially affects the differentiation of naïve T cells to Th1 and Th17 effector T cells in dLN. This possibility was supported by our MOG tetramer experiment, in which we observed a significant reduction in the number of MOG-specific CD4 + T cells in the dLN.
Our RNA sequencing analysis further showed that the expression of various genes involved in EAE pathogenesis was significantly downregulated in dLN by mAb SF1 administration. These include the signal transduction and transcription activator Stat3 , and cytokines and their receptors Il17a , Il17f , Il22 , and Ifngr1 , suggesting a central role of 6-sulfo sLe X in reducing the numbers of lymphocytes in the dLN, leading to the onset of clinical symptoms of EAE. The downregulation of Stat3 expression aligns with previous studies in which Stat3 was found to play a core role in the differentiation and function of Th17 cells ( 27 ). In addition, Il17f and Il17a , related to Th17 cells, are involved in promoting the inflammatory process; Il6 and stat3 , key factors in the differentiation of Th17 cells, play a significant role in exacerbating early inflammatory responses and disease progression in EAE; and Il22 may aid in protecting the CNS from EAE-related inflammatory damage. The decreased expression of these factors may impair the ability of lymphocytes to differentiate in the dLN, thereby affecting the number of lymphocytes in lesion areas in the CNS ( 33 , 34 ). Furthermore, our bioinformatics analyses, which included GO and GSEA signaling pathway analyses, showed that pathways related to the inflammatory response, IFN-γ response, IL2/STAT5 signaling, and PI3K/AKT/mTOR signaling were significantly suppressed by SF1 administration in the dLN. These results further emphasize the crucial role of 6-sulfo sLe X in the regulation of multiple aspects of lymphocyte activation and the inflammatory response in EAE.
The initial attachment of naive T cells to the surface of HEV, mediated by slow rolling via l -selectin (CD62L) and 6-sulfo sLe X , leads to downstream signaling activation of PI3Kσ, resulting in the shedding of l -selectin from naive T cells and a concurrent downregulation of l -selectin gene transcription. This process drives the migration of Tem cells from secondary lymphoid organs to peripheral tissues, marking the transition from naive T cells to effector T cells ( 35 , 36 ). The lymphocyte-homing receptor l -selectin reportedly plays a crucial role in promoting EAE by facilitating the adhesion and activation of inflammatory leukocytes ( 37 , 38 ). In contrast, another study showed that l -selectin-deficient mice developed a severe EAE comparable to that of WT mice ( 39 ), rendering the role of l -selectin in EAE pathogenesis controversial. Therefore, such an antibody-drug would cause severe side effects. In contrast, 6-sulfo sLe X glycans are specifically expressed in the HEV, and no other tissues or organs express this glycan ( 21 ), suggesting that this glycan may serve as an ideal target for drug development. Another anti-HEV antibody, MECA-79, which recognizes O -glycans containing 6-sulfo N -acetylglucosamine in the extended core 1 structure and partially shares the glycan epitope recognized by SF1 ( 40 ), has been effectively used in HEV-labeling experiments ( 41–44 ). However, to the best of our knowledge, no study has examined the therapeutic application of MECA-79 in EAE. Therefore, the SF1 antibody targeting 6-sulfo sLe X represents a novel and promising strategy against MS.
Several innovative treatments for MS have been developed, but they may trigger serious adverse effects. Although effective, fingolimod is associated with several adverse effects, including cardiac arrhythmia and fingolimod-associated macular edema ( 45 ). Natalizumab, an antibody-drug against α4 integrin, is linked to an increased risk of progressive multifocal leukoencephalopathy ( 46 ). Alemtuzumab can improve clinical and radiological outcomes but can also lead to severe autoimmune diseases, including thyroid disease and thrombocytopenia ( 47 ). Another contemporary MS drug, ocrelizumab, is associated with an increased risk of severe infection ( 48 ). In contrast, our proposed strategy using mAb SF1, owing to its high specificity, predominantly binds to glycan ligands on the surface of HEV in the PLN, potentially posing fewer side effects. In WT mice, administration of SF1 over a period of 5 days did not elicit any significant alterations in the lymphocyte counts in both the blood and spleen, compared with the PBS group ( Supplementary Fig. 5 ). In addition, a long-term administration of SF1 antibody to the C57BL/6 mice for 16-weeks, the appearance, movement, water and food intake, and the body weight were normal ( Supplementary Fig. 8A, B ). Furthermore, histological examination of various organs including the brain, heart, colon, small intestine, kidney, liver, lung, pancreas, spleen, and thymus by hematoxylin-eosin staining indicated normal morphology without any signs of inflammation or any other side effects ( Supplementary Fig. 8C ).
Our study sheds new light on the role of 6-sulfo sLe X glycan in EAE pathogenesis. Future work should explore the role of this unique sulfated glycan in other animal models of immune-related diseases, broadening the scope and application of our current findings because our research provides a theoretical basis for the development of potential therapeutics against various immune-related diseases targeting glycan-mediated immune cell trafficking. One limitation of this study is that we found the preventive but not therapeutic effects of mAb SF1 because of the technical difficulties to observe consistent relapsing phase in the EAE model used in this study. Nevertheless, we believe that even the preventive approach taken in this study has provided a valuable basis for the potential application of mAb SF1 in MS treatment in the future. In conclusion, our study revealed the potential of the sulfated glycan, 6-sulfo sLe x , as a novel target for MS.
We thank Dr Wei Liu, Mr Hanlin Yang, and Dr Wenxin Liu for their helpful discussions and technical assistance. We would like to express our sincere gratitude to Dr Minoru Fukuda at the Sanford Burnham Prebys Medical Discovery Institute for generously providing GlcNAc6ST-1/2 duoble knockout mice and for granting us permission to use them in this study.
Q.L.: Investigation, Data curation, Writing—original draft. W.X.: Investigation, Writing—review & editing. S.O.: Investigation, Writing—review & editing. H.A.: Supervision, Writing—review & editing. H.N.: Supervision, Writing—review & editing. H.K.: Conceptualization, Funding acquisition, Supervision, Writing—review & editing.
Conflict of interest statement . H.K. is the inventor of a PCT patent application (PCT/JP2021/036893) regarding the development and application of the SF1 antibody led by Chiba University. The other authors declare that they have no competing interests regarding the contents of this article.
This research was partly supported by the Japan Agency for Medical Research and Development (AMED; grant numbers 20ae0101034h0005, 22ae0121017h0002, and 22ym0126065h0001), JSPS KAKENHI (grant numbers 20H03379 and 20K07541), and the Institute for Advanced Academic Research at Chiba University.
The dataset [PRJNA977076] used in this study can be found in the GEO repository. Further inquiries can be directed to the corresponding authors.
Dendrou A , Fugger L , Friese MA. Immunopathology of multiple sclerosis . Nat Rev Immunol 2015 ; 15 : 545 – 58 . https://doi.org/10.1038/nri3871
Google Scholar
McGinley MP , Cohen JA. Sphingosine 1-phosphate receptor modulators in multiple sclerosis and other conditions . Lancet 2021 ; 398 : 1184 – 94 . https://doi.org/10.1016/S0140-6736(21)00244-0
Cencioni MT , Mattoscio M , Magliozzi R , et al. . B cells in multiple sclerosis—from targeted depletion to immune reconstitution therapies . Nat Rev Neurol 2021 ; 17 : 399 – 414 . https://doi.org/10.1038/s41582-021-00498-5
Yong HYF , Yong VW. Mechanism-based criteria to improve therapeutic outcomes in progressive multiple sclerosis . Nat Rev Neurol 2022 ; 18 : 40 – 55 . https://doi.org/10.1038/s41582-021-00581-x
Constantinescu CS , Farooqi N , O’Brien K , et al. . Experimental autoimmune encephalomyelitis (EAE) as a model for multiple sclerosis (MS) . Br J Pharmacol 2011 ; 164 : 1079 – 106 . https://doi.org/10.1111/j.1476-5381.2011.01302.x
Kleine TO , Benes L. Immune surveillance of the human central nervous system (CNS): different migration pathways of immune cells through the blood–brain barrier and blood-cerebrospinal fluid barrier in healthy persons . J Neuroimmunol 2006 ; 69A : 147 – 51 . https://doi.org/10.1002/cyto.a.20225
Bettelli E , Sullivan B , Szabo SJ , et al. . Loss of T-bet, but not STAT1, prevents the development of experimental autoimmune encephalomyelitis . J Exp Med 2004 ; 200 : 79 – 87 . https://doi.org/10.1084/jem.20031819
Ivanov II , McKenzie BS , Zhou L , et al. . The orphan nuclear receptor RORgammat directs the differentiation program of proinflammatory IL-17+ T helper cells . Cell 2006 ; 126 : 1121 – 33 . https://doi.org/10.1016/j.cell.2006.07.035
Lee HG , Lee JU , Kim DH , et al. . Pathogenic function of bystander-activated memory-like CD4+ T cells in autoimmune encephalomyelitis . Nat Commun 2019 ; 10 : 709 . https://doi.org/10.1038/s41467-019-08482-w
Sun D , Whitaker JN , Huang Z , et al. . Myelin antigen-specific CD8+ T cells are encephalitogenic and produce severe disease in C57BL/6 mice . J Immunol 2001 ; 166 : 7579 – 87 . https://doi.org/10.4049/jimmunol.166.12.7579
Wagner CA , Roqué PJ , Mileur TR , et al. . Myelin-specific CD8+ T cells exacerbate brain inflammation in CNS autoimmunity . J Clin Invest 2020 ; 130 : 203 – 13 . https://doi.org/10.1172/JCI132531
Jacobsen M , Cepok S , Quak E , et al. . Oligoclonal expansion of memory CD8+ T cells in cerebrospinal fluid from multiple sclerosis patients . Brain 2002 ; 125 : 538 – 50 . https://doi.org/10.1093/brain/awf059
Jilek S , Schluep M , Rossetti AO , et al. . CSF enrichment of highly differentiated CD8+ T cells in early multiple sclerosis . Clin Immunol 2007 ; 123 : 105 – 13 . https://doi.org/10.1016/j.clim.2006.11.004
Okada T , Cyster JG. B cell migration and interactions in the early phase of antibody responses . Curr Opin Immunol 2006 ; 18 : 278 – 85 . https://doi.org/10.1016/j.coi.2006.02.005
Cyster JG , Allen CDC. B cell responses: cell interaction dynamics and decisions . Curr Opin Immunol 2021 ; 24 : 332 – 6 . https://doi.org/10.1016/j.cell.2019.03.016
Kansas GS. Selectins and their ligands: current concepts and controversies . Blood 1996 ; 88 : 3259 – 87 . https://doi.org/10.1182/blood.V88.9.3259.bloodjournal8893259
Allen CD , Okada T , Tang HL , et al. . Imaging of germinal center selection events during affinity maturation . Science 2007 ; 315 : 528 – 31 . https://doi.org/10.1126/science.1136736
Hiraoka N , Petryniak B , Nakayama J , et al. . A novel, high endothelial venule-specific sulfotransferase expresses 6-sulfo sialyl Lewis(x), an l -selectin ligand displayed by CD34 . Immunity 1999 ; 11 : 79 – 89 . https://doi.org/10.1016/s1074-7613(00)80083-7
Uchimura K , Gauguet JM , Singer M , et al. . A major class of l -selectin ligands is eliminated in mice deficient in two sulfotransferases expressed in high endothelial venules . Nat Immunol 2005 ; 6 : 1105 – 13 . https://doi.org/10.1038/ni1258
Kawashima H , Petryniak B , Hiraoka N , et al. . N -acetylglucosamine-6- O -sulfotransferases 1 and 2 cooperatively control lymphocyte homing through l -selectin ligand biosynthesis in high endothelial venules . Nat Immunol 2005 ; 6 : 1096 – 104 . https://doi.org/10.1038/ni1259
Liu W , Xiong W , Liu W , et al. . A novel monoclonal antibody against 6-sulfo sialyl Lewis X glycans attenuates murine allergic rhinitis by suppressing Th2 immune responses . Sci Rep 2023 ; 13 : 15740 . https://doi.org/10.1038/s41598-023-43017-w
van Zwam M , Huizinga R , Heijmans N , et al. . Surgical excision of CNS-draining lymph nodes reduces relapse severity in chronic-relapsing experimental autoimmune encephalomyelitis . J Pathol 2009 ; 217 : 543 – 51 . https://doi.org/10.1002/path.2476
Bolton C , Wood EG , Ayoub SS. N -methyl- d -aspartate (NMDA) receptor involvement in central nervous system prostaglandin production during the relapse phase of chronic relapsing experimental autoimmune encephalomyelitis (CR EAE) . Fundam Clin Pharmacol 2013 ; 27 : 535 – 43 . https://doi.org/10.1111/j.1472-8206.2012.01050.x
Laman JD , Weller RO. Drainage of cells and soluble antigen from the CNS to regional lymph nodes . J Neuroimmune Pharmacol 2013 ; 8 : 840 – 56 . https://doi.org/10.1007/s11481-013-9470-8
Ryu JK , Petersen MA , Murray SG , et al. . Blood coagulation protein fibrinogen promotes autoimmunity and demyelination via chemokine release and antigen presentation . Nat Commun 2015 ; 6 : 8164 . https://doi.org/10.1038/ncomms9164
Weidemüller P , Kholmatov M , Petsalaki E , et al. . Transcription factors: bridge between cell signaling and gene regulation . Proteomics 2021 ; 21 : e2000034 . https://doi.org/10.1002/pmic.202000034
Liu X , Lee YS , Yu CR , et al. . Loss of STAT3 in CD4+ T cells prevents development of experimental autoimmune diseases . J Immunol 2008 ; 180 : 6070 – 6 . https://doi.org/10.4049/jimmunol.180.9.6070
McGinley AM , Sutton CE , Edwards SC , et al. . Interleukin-17A serves a priming role in autoimmunity by recruiting IL-1β-producing myeloid cells that promote pathogenic T cells . Immunity 2020 ; 52 : 342 – 356.e6 . https://doi.org/10.1016/j.immuni.2020.01.002
Ueno R , Miyamoto K , Tanaka N , et al. . Keratan sulfate exacerbates experimental autoimmune encephalomyelitis . J Neurosci Res 2015 ; 93 : 1874 – 80 . https://doi.org/10.1002/jnr.23640
Hiraoka N , Kawashima H , Petryniak B , et al. . Core 2 branching beta1,6- N -acetylglucosaminyltransferase and high endothelial venule-restricted sulfotransferase collaboratively control lymphocyte homing . J Biol Chem 2004 ; 279 : 3058 – 67 . https://doi.org/10.1074/jbc.M311150200
Tostanoski LH , Chiu YC , Gammon JM , et al. . Reprogramming the local lymph node microenvironment promotes tolerance that is systemic and antigen specific . Cell Rep 2016 ; 16 : 2940 – 52 . https://doi.org/10.1016/j.celrep.2016.08.033
Butcher EC , Picker LJ. Lymphocyte homing and homeostasis . Science 1996 ; 272 : 60 – 6 . https://doi.org/10.1126/science.272.5258.60
Mebius RE. Organogenesis of lymphoid tissues . Nat Rev Immunol 2003 ; 3 : 292 – 303 . https://doi.org/10.1038/nri1054
O’Shea JJ , Murray PJ. Cytokine signaling modules in inflammatory responses . Immunity 2008 ; 28 : 477 – 87 . https://doi.org/10.1016/j.immuni.2008.03.002
Galkina E , Tanousis K , Preece G , et al. . l -Selectin shedding does not regulate constitutive T cell trafficking but controls the migration pathways of antigen-activated T lymphocytes . J Exp Med 2003 ; 198 : 1323 – 35 . https://doi.org/10.1084/jem.20030485
Venturi GM , Tu L , Kadono T , et al. . Leukocyte migration is regulated by l -selectin endoproteolytic release . Immunity 2003 ; 19 : 713 – 24 . https://doi.org/10.1016/s1074-7613(03)00295-4
Li O , Liu JQ , Zhang H , et al. . CD62L is required for the priming of encephalitogenic T cells but does not play a major role in the effector phase of experimental autoimmune encephalomyelitis . Scand J Immunol 2006 ; 64 : 117 – 24 . https://doi.org/10.1111/j.1365-3083.2006.01783.x
Grewal IS , Foellmer HG , Grewal KD , et al. . CD62L is required on effector cells for local interactions in the CNS to cause myelin damage in experimental allergic encephalomyelitis . Immunity 2001 ; 14 : 291 – 302 . https://doi.org/10.1016/s1074-7613(01)00110-8
Uboldi C , Döring A , Alt C , et al. . l -selectin-deficient SJL and C57BL/6 mice are not resistant to experimental autoimmune encephalomyelitis . Eur J Immunol 2008 ; 38 : 2156 – 67 . https://doi.org/10.1002/eji.200838209
Yeh JC , Hiraoka N , Petryniak B , et al. . Novel sulfated lymphocyte homing receptors and their control by a Core1 extension beta 1,3- N -acetylglucosaminyltransferase . Cell 2001 ; 105 : 957 – 69 . https://doi.org/10.1016/s0092-8674(01)00394-4
Streeter PR , Rouse BT , Butcher EC. Immunohistologic and functional characterization of a vascular addressin involved in lymphocyte homing into peripheral lymph nodes . J Cell Biol 1988 ; 107 : 1853 – 62 . https://doi.org/10.1083/jcb.107.5.1853
Suzawa K , Kobayashi M , Sakai Y , et al. . Preferential induction of peripheral lymph node addressin on high endothelial venule-like vessels in the active phase of ulcerative colitis . Am J Gastroenterol 2007 ; 102 : 1499 – 509 . https://doi.org/10.1111/j.1572-0241.2007.01189.x
Martinet L , Garrido I , Filleron T , et al. . Human solid tumors contain high endothelial venules: association with T- and B-lymphocyte infiltration and favorable prognosis in breast cancer . Cancer Res 2011 ; 71 : 5678 – 87 . https://doi.org/10.1158/0008-5472.CAN-11-0431
Menzel L , Zschummel M , Crowley T , et al. . Lymphocyte access to lymphoma is impaired by high endothelial venule regression . Cell Rep 2021 ; 37 : 109878 . https://doi.org/10.1016/j.celrep.2021.109878
Mandal P , Gupta A , Fusi-Rubiano W , et al. . Fingolimod: therapeutic mechanisms and ocular adverse effects . Eye (Lond) 2017 ; 31 : 232 – 40 . https://doi.org/10.1038/eye.2016.258
Berger JR. Natalizumab and progressive multifocal leucoencephalopathy . Ann Rheum Dis 2006 ; 65 : iii48 – 53 . https://doi.org/10.1136/ard.2006.058404
Coles AJ , Twyman CL , Arnold DL , et al. ; CARE-MS II investigators . Alemtuzumab for patients with relapsing multiple sclerosis after disease-modifying therapy: a randomised controlled phase 3 trial . Lancet 2012 ; 380 : 1829 – 39 . https://doi.org/10.1016/S0140-6736(12)61768-1
Hauser SL , Bar-Or A , Comi G , et al. ; OPERA I and OPERA II Clinical Investigators . Ocrelizumab versus Interferon Beta-1a in relapsing multiple sclerosis . N Engl J Med 2017 ; 376 : 221 – 34 . https://doi.org/10.1056/NEJMoa1601277
- systemic lupus erythematosus
- monoclonal antibodies
- experimental autoimmune encephalomyelitis
- lymphocytes
- multiple sclerosis
- polysaccharides
- sierra leone
- t-lymphocytes
- spinal cord
- lymphocyte migration into lymph node
- sialyl lewis x antigen
Supplementary data
Email alerts, companion article.
- In This Issue
Citing articles via
- X (formerly Twitter)
- Recommend to your Library
Affiliations
- Online ISSN 1460-2377
- Copyright © 2024 Japanese Society for Immunology
- About Oxford Academic
- Publish journals with us
- University press partners
- What we publish
- New features
- Open access
- Institutional account management
- Rights and permissions
- Get help with access
- Accessibility
- Advertising
- Media enquiries
- Oxford University Press
- Oxford Languages
- University of Oxford
Oxford University Press is a department of the University of Oxford. It furthers the University's objective of excellence in research, scholarship, and education by publishing worldwide
- Copyright © 2024 Oxford University Press
- Cookie settings
- Cookie policy
- Privacy policy
- Legal notice
This Feature Is Available To Subscribers Only
Sign In or Create an Account
This PDF is available to Subscribers Only
For full access to this pdf, sign in to an existing account, or purchase an annual subscription.
An official website of the United States government
Official websites use .gov A .gov website belongs to an official government organization in the United States.
Secure .gov websites use HTTPS A lock ( Lock Locked padlock icon ) or https:// means you've safely connected to the .gov website. Share sensitive information only on official, secure websites.
- Publications
- Account settings
- Advanced Search
- Journal List

Cnp Promoter-Driven Sustained ERK1/2 Activation Increases B-Cell Activation and Suppresses Experimental Autoimmune Encephalomyelitis
Marisa a jeffries, alison e obr, kelly urbanek, sharyl l fyffe-maricich, teresa l wood.
- Author information
- Article notes
- Copyright and License information
Sharyl L. Fyffe-Maricich is presently affiliated with Ultragenyx Pharmaceutical, Novato, CA 94949, United States.
Sharyl L. Fyffe-Maricich, Ultragenyx Pharmaceutical, 60 Leveroni Ct., Novato, CA 94949, United States. Email: [email protected]
Teresa L. Wood, Rutgers New Jersey Medical School, 205 S. Orange Ave., Cancer Center H1200, Newark, NJ 07103, United States. Email: [email protected]
Received 2020 Jul 13; Revised 2020 Sep 29; Accepted 2020 Oct 9; Collection date 2020 Jan-Dec.
Creative Commons Non Commercial CC BY-NC: This article is distributed under the terms of the Creative Commons Attribution-NonCommercial 4.0 License ( https://creativecommons.org/licenses/by-nc/4.0/ ) which permits non-commercial use, reproduction and distribution of the work without further permission provided the original work is attributed as specified on the SAGE and Open Access pages ( https://us.sagepub.com/en-us/nam/open-access-at-sage ).
The ERK1/2 signaling pathway promotes myelin wrapping during development and remyelination, and sustained ERK1/2 activation in the oligodendrocyte (OL) lineage results in hypermyelination of the CNS. We therefore hypothesized that increased ERK1/2 signaling in the OL lineage would 1) protect against immune-mediated demyelination due to increased baseline myelin thickness and/or 2) promote enhanced remyelination and thus functional recovery after experimental autoimmune encephalomyelitis (EAE) induction. Cnp-Cre;Mek1DD-eGFP/+ mice that express a constitutively active form of MEK1 (the upstream activator of ERK1/2) in the OL lineage, exhibited a significant decrease in EAE clinical severity compared to controls. However, experiments using tamoxifen-inducible Plp-Cre ERT ;Mek1DD-eGFP/+ or Pdgfrα-Cre ERT ;Mek1DD-eGFP mice revealed this was not solely due to a protective or reparative effect resulting from MEK1DD expression specifically in the OL lineage. Because EAE is an immune-mediated disease, we examined Cnp-Cre ; Mek1DD-eGFP/+ splenic immune cells for recombination. Surprisingly, GFP + recombined CD19 + B-cells, CD11b + monocytes, and CD3 + T-cells were noted when Cre expression was driven by the Cnp promoter. While ERK1/2 signaling in monocytes and T-cells is associated with proinflammatory activation, fewer studies have examined ERK1/2 signaling in B-cell populations. After in vitro stimulation, MEK1DD-expressing B-cells exhibited a 3-fold increase in CD138 + plasmablasts and a 5-fold increase in CD5 + CD1d hi B-cells compared to controls. Stimulated MEK1DD-expressing B-cells also exhibited an upregulation of IL-10, known to suppress the initiation of EAE when produced by CD5 + CD1d hi regulatory B-cells. Taken together, our data support the conclusion that sustained ERK1/2 activation in B-cells suppresses immune-mediated demyelination via increasing activation of regulatory B10 cells.
Keywords: B10-cell, B-cell, demyelination, EAE, oligodendrocyte, ERK1/2
Myelination, once thought to be a passive insulation surrounding axons, is now known to play an active role in modulating central nervous system (CNS) function. In addition to optimizing conduction velocity, oligodendrocytes (OLs), the myelinating cells of the CNS, provide trophic and metabolic support to axons without which axons degenerate ( Funfschilling et al., 2012 ; Simons & Nave, 2015 ; Meyer et al., 2018 ). In demyelinating diseases such as multiple sclerosis (MS), autoimmune-mediated loss of myelin and oligodendrocytes (OLs) in the central nervous system (CNS) results in damage to neurons causing a progressive decline in patient mobility. Current immunosuppression treatments do not adequately prevent demyelination and disease progression. Additionally, no therapeutic options exist that target OLs to increase resistance to immune attack or promote myelin repair. Therefore, additional research to elucidate mechanisms of limiting demyelination and stimulating remyelination is critical to increase therapeutic efficacy.
Previous studies have examined the role of extracellular signal-regulated kinases 1 and 2 (ERK1/2) in developmental myelination and remyelination ( Ishii et al., 2012 ; Fyffe-Maricich et al., 2013 ; Ishii et al., 2013 ; Michel et al., 2015 ; Jeffries et al., 2016 ). Of particular importance are data revealing that ERK1/2 signaling critically promotes timely myelin repair and can regulate remyelination thickness after lysophosphatidylcholine (LPC) injection, a detergent that disrupts lipid-rich myelin membranes leading to focal demyelination. When ERK2 is conditionally deleted in the OL lineage, this results in delayed myelin wrapping after LPC demyelination of the corpus callosum ( Michel et al., 2015 ). Conversely, sustained ERK1/2 activation in the OL lineage leads to enhanced myelin thickness during remyelination after LPC injection into the spinal cord without affecting demyelination ( Fyffe-Maricich et al., 2013 ). Surprisingly, sustained ERK1/2 activation specifically in mature OLs prior to demyelination results in MEK1DD-expressing pre-existing OLs that persist in a LPC lesion and form myelin basic protein (MBP) positive processes that may contribute to remyelination, which normally does not occur ( Jeffries et al., 2016 ). Taken together, these studies have highlighted ERK1/2 signaling in the OL lineage as a key regulator of remyelination, particularly with regards to myelin thickness. However, to date no studies have examined ERK1/2 signaling in the OL lineage in an immune-mediated model of demyelination like experimental autoimmune encephalomyelitis (EAE), which recapitulates the immune component of MS pathology.
In order to determine whether sustained ERK1/2 activation in the OL lineage results in decreased EAE severity, we examined Cnp-Cre;Mek1DD-eGFP/+ (Cnp-Mek1DD) mice that express constitutively-active MEK1, the upstream kinase of ERK1/2, under the Cnp promoter which drives expression in the OL lineage in the CNS and Schwann cells in the peripheral nervous system (PNS). Previous studies using this mouse line have revealed hypermyelination throughout the CNS and PNS ( Fyffe-Maricich et al., 2013 ; Ishii et al., 2013 ). In particular, Cnp-Mek1DD mice exhibited increased remyelination thickness after LPC demyelination of the spinal cord ( Fyffe-Maricich et al., 2013 ). Because these mice exhibit hypermyelination during myelin repair after LPC injection, we hypothesized that we might similarly see a beneficial effect of ERK1/2 signaling in the context of EAE. Extraordinarily, we found that Cnp-Mek1DD mice did not develop severe EAE symptoms like their control littermates, suggesting a protective effect against EAE induction. To validate these results we used a tamoxifen-inducible Cre-lox mouse model, Plp-Cre ERT ;Mek1DD-eGFP/+ (Plp-Mek1DD) that expresses MEK1DD in mature OLs only after exposure to tamoxifen. Plp-Mek1DD mice that were given tamoxifen 40 days prior to EAE induction exhibited a disease course similar to control mice, indicating that sustained ERK1/2 activation in mature OLs, and the accompanying increased myelin thickness, does not protect against EAE. In order to examine whether Cnp-Mek1DD mice had attenuated EAE disease course due to recombined oligodendrocyte progenitor cells (OPCs) responsible for remyelination, we administered tamoxifen to Pdgfrα-Cre ERT ;Mek1DD-eGFP (Pdgfrα-Mek1DD) mice during EAE. However, these mice did not exhibit improved functional scores during EAE compared to controls, suggesting that sustained ERK1/2 activation in adult OPCs during remyelination does not improve function in the EAE model. Upon further examination, we discovered that the Cnp promoter was inducing recombination in splenic immune cell populations; namely, CD19 + B-cells, CD11b + monocytes, and CD3 + T-cells. Isolation of CD19 + splenic B-cells from Cnp-Mek1DD and control mice followed by in vitro stimulation with lipopolysaccharides (LPS) resulted in enhanced B-cell activation. In particular, enriched expansion of the CD5 + CD1d hi population and increased IL-10 transcription and secretion, associated with regulatory B10 cells, was observed. Taken together, our data reveal that the Cnp promoter is active not only in the OL lineage of the CNS, but also in splenic B-cells, monocytes, and T-cells. Furthermore, expression of constitutively active MEK1 in splenic B-cells results in increased activation, particularly for IL-10 producing regulatory B10 cells. The results from this study suggest that enhancing B10 cell activation and function through downstream effectors of ERK1/2 signaling may be of therapeutic interest in preventing progressive damage in immune-mediated demyelination disorders such as MS.
Materials and Methods
Experimental animals.
Heterozygous Cnp-Cre ( Lappe-Siefke et al., 2003 ), Plp-Cre ERT ( Doerflinger et al., 2003 ) (The Jackson Laboratory, RRID:ISMR_JAX:005975 ), or Pdgfrα-Cre ERT ( Kang et al., 2010 ) (The Jackson Laboratory, RRID:ISMR_JAX:018280 ) mice on a C57Bl/6 background were bred to homozygous R26Stop FL Mek1DD-eGFP mice ( Srinivasan et al., 2009 ) (The Jackson Laboratory; RRID:ISMR_JAX:012352 ) in order to generate female Cnp-Cre;Mek1DD-eGFP/+ (Cnp-Mek1DD), Plp-Cre ERT ;Mek1DD-eGFP/+ (Plp-Mek1DD), or Pdgfrα-Cre ERT ;Mek1DD-eGFP/+ (Pdgfrα-Mek1DD) mice and control littermates ( WT;Mek1DD-eGFP/+ ). In the Cnp-Mek1DD mouse CNS, the OL lineage exhibits recombination and expression of MEK1DD, a constitutively active MEK1 mutant rat MAPKK1 (the ERK1/2 kinase) with two serine to aspartic acid substitutions (S218D/S222D) within the catalytic domain. For some experiments, Cnp-Cre or Plp-Cre ERT mice were crossed with ROSA mT/mG double reporter (DR) ( Muzumdar et al., 2007 ) (The Jackson Laboratory; RRID:ISMR_JAX:007676 ) mice to generate Cnp-Cre; ROSA mT/mG (Cnp-DR) or Plp-Cre ERT ;ROSA mT/mG (Plp-DR) mice that exhibit widespread membrane-bound TdTomato expression but upon Cre recombination express membrane-bound GFP in recombined cells. In order to induce recombination in Plp-Mek1DD, Plp-DR, or Pdgfrα-Mek1DD mice, tamoxifen (100 mg/kg; 10 mg/mL stock in 9:1 sunflower seed oil:ethanol) was administered by intraperitoneal injection for 5 consecutive days, resulting in the recombination in PLP + mature OLs or PDGFRα + OPCs respectively. In Cnp-Mek1DD and Plp-Mek1DD mice, expression of MEK1DD is concurrent with an eGFP marker downstream of an internal ribosomal entry site, such that recombined cells can be identified. Mice were kept in microisolation in a pathogen-free environment and maintained on a 12 h light:12 h dark cycle with standard mouse chow and water ad libitum . All research and animal care procedures were approved by the University of Pittsburgh Institutional Animal Care and Use Committee or the Rutgers New Jersey Medical School Institutional Animal Care and Use Committee.
Experimental Autoimmune Encephalomyelitis
In order to induce immune-mediated demyelination of the CNS in Cnp-Mek1DD and control littermates, 10-14 week old female mice were given EAE according to the MOG 35-55 peptide EAE kit (EK-2110, Hooke Laboratories). To induce recombination before EAE, 6-11 week old Plp-Mek1DD and control female mice were administered tamoxifen (10 mg/mL; T5648, Sigma) dissolved in 9:1 sunflower seed oil and ethanol by intraperitoneal injection at 100 mg/kg once daily for 5 consecutive days. The first injection was given 40 days prior to EAE induction at 12-17 weeks of age in order to allow for significant hypermyelination, observed previously by 21-60 days post-tamoxifen (dpt) ( Jeffries et al., 2016 ). Pdgfrα-Mek1DD and control littermate mice were given EAE at 10-12 weeks of age. Each mouse received tamoxifen injections as described above once daily for 5 consecutive days once a clinical score of 1 was reached. When mice reached a paralyzed state during EAE, they received softened wet mouse chow ad libitum and were checked daily for signs of dehydration and weight loss. If mice exhibited dehydration, they were administered 500 µL of 0.9% saline subcutaneously once daily. Only female mice were used in all experiments as EAE induction in males results in higher unpredictability and variability in disease.
Luxol Fast Blue Staining of Frozen Sections
Mice were intracardially perfused with 1× PBS followed by 4% PFA/PBS, post-fixed for 2–24 hours, then cryoprotected overnight in 20% sucrose. Fixed spinal cord tissue was embedded and cryosectioned at 20 µm sections. Sections were then rinsed in water followed by 35% and 70% EtOH, then incubated in luxol fast blue solution overnight at 55-60°C. Excess stain was rinsed with 95% EtOH followed by water, then destaining was performed in 0.05% lithium carbonate solution before additional 70% and 100% EtOH rinsing followed by xylene immersion and coverslipping.
Immunolabeling of Frozen Sections
Mice were intracardially perfused with 1× PBS followed by 4% PFA/PBS, post-fixed for 2-24 hours, then cryoprotected overnight in 20% sucrose. Fixed spinal cord tissue was embedded and cryosectioned at 20 µm sections. Immunolabeling was performed as described previously in ( Jeffries et al., 2016 ) with slight modifications. Briefly, for MBP immunofluorescence, sections were rehydrated in 1× PBS then submerged in 100% EtOH for 10 minutes (min). After 3 washes in 1× PBS, sections were blocked in 2-4% NGS/0.3% Triton-X-100 in 1× PBS, then incubated with primary antibodies diluted in blocking buffer at 4°C overnight. After primary antibody incubation, sections were washed 3x 5 min. in 1× PBS followed by secondary antibodies at room temperature for 1-3 hours. Primary antibodies used were mouse anti-MBP (1:1000; SMI-99, Covance, RRID:AB_2314772 ), rabbit anti-IBA1 (1:500; 019-19741, Wako, RRID:AB_839504 ), rat anti-CD3 (1:50; MAB4841, R&D Systems, RRID:AB_358426 ), and rabbit anti-CD19 (1:1000; 90176, Cell Signaling, RRID:AB_2800152 ). Secondary antibodies used were goat anti-mouse 488 (1:500; A11001, Invitrogen, RRID:AB_2534069 ), goat anti-rabbit 546 (1:500; A11010, Invitrogen, RRID:2534077 ), goat anti-rat 350 (1:500; A21093, Invitrogen, RRID:AB_2535748 ), goat anti-rabbit 647 (1:500; A21246, Invitrogen, RRID:AB_2535814 ). Sections were counterstained with DAPI (1:1000) in order to identify demyelinated lesions by hypercellularity. Slides were coverslipped with Fluorogel (17985-10, Electron Microscopy Sciences) or Prolong Gold ( P36934 , Thermofisher Scientific).
Toluidine Blue Sections
Mice were intracardially perfused with 4% PFA, then spinal cords were dissected and drop-fixed in 4% PFA/2% glutaraldehyde in 0.1 M sodium cacodylate buffer for ≥ 24 hrs. Tissue samples were postfixed in 1% OsO 4 , then dehydrated in serial ethanol solutions, stained en bloc with uranyl acetate, and embedded in a Poly/Bed812 resin. Thick (350 nm) sections were cut and stained with toluidine blue and visualized at 60x magnification.
Image Analysis
Fluorescence and brightfield images were acquired using an Olympus AX-70 microscope and iVision software. Immunofluorescence images for analysis were acquired using a 2x objective. Toluidine blue ventral spinal cord white matter sections were imaged using a 60x oil objective. Imaged areas were selected for sparse or no visible immune infiltration and demyelination. Luxol fast blue images were acquired using a 2x objective. CD3 and CD19 immunostaining images were acquired using a 10x objective. For MBP and IBA1 positivity in the white matter of the spinal cord, the dorsal and ventral white matter regions were outlined as the region of interest (ROI). Images for analyzed using the adjust threshold function for positive staining and area fraction within the ROI was calculated using ImageJ software. At least 2 images per animal and 3 animals per genotype were analyzed.
Spleen Dissociation
Female Cnp-Mek1DD, Cnp-DR, or Plp-DR and control littermate mice were euthanized by CO 2 inhalation at 8-13 weeks of age. Spleens were removed and immediately placed in 2 mL DMEM on ice, and dissociated as described previously with modifications ( Pachynski et al., 2015 ). Spleens were chopped and 1 mL of digestion medium in HBSS added (0.04 mg/mL collagenase I, LS004214 , Worthington Biochemical; 1.67 mg/mL collagenase IV, LS004188 , Worthington Biochemical; 30 mg/mL DNAse I, D4527-40KU, Sigma) for 25 min. incubation at 37°C, then 5 mL of DMEM were added and suspension filtered through a 70 µm filter. Cells were centrifuged at 450 g for 6 min., then erythrocytes were lysed using 8.29 g/L NH 4 Cl, 1 g/L KHCO 3 , and 37.2 mg/L EDTA for 4 min. After adding 12 mL of DMEM, suspensions were filtered through a 70 µm filter, washed with 10 mL DMEM, and centrifuged at 450 g for 6 min. Cells were resuspended in 20 mL of HBSS, centrifuged at 450 g for 5 min., then resuspended in 1 mL HBSS for cell counting with trypan blue. After cell counting with a 1:4 dilution, cells were centrifuged at 450 g for 5 min. and resuspended at 1 x 10 6 cells/50 uL of FACS buffer (2% goat serum, G9023, Sigma; 1% BSA, A6003, Sigma; in HBSS). Only female mice were used for all immune cell experiments in order to match EAE experiments.
Splenic Flow Cytometry
Resuspended splenic immune cells in FACS buffer were immunostained using appropriate combinations of the following antibodies in 50 uL FACS buffer/sample: anti-CD45-PE/Cy5 (103109, RRID:AB_312974 ), anti-CD19-PE/Cy7 (115520, RRID:AB_313655 ), anti-CD3-BV510 (100233, RRID:AB_2561387 ), anti-CD4-APC (100411, RRID:AB_312696 ), anti-CD8a-PE (100707, RRID:AB_312746 ), anti-CD11b-BV605 (101237, RRID:AB_11126744 ), anti-MHCII-APC/Cy7 (107627, RRID:AB_1659252 ), anti-CD5-PE/Cy5 (100609, RRID:AB_312738 ), anti-CD1d-PE (123509, RRID:AB_1236547 ), anti-CD138-BV510 (142521, RRID:AB_2562727 ). All antibodies were obtained from BioLegend (San Diego, CA). Cells were incubated in primary antibodies for 40 min. in the dark, followed by the addition of 50 µL of heat-inactivated FBS (F0926, Sigma) and centrifugation. For GFP+ recombination experiments, PacBlue Live/Dead staining ( L34955 , Invitrogen) was performed to verify viability in addition to trypan blue cell counts prior to staining to assess viability. For all analyses, cells were fixed with 1% PFA (15714-S, Electron Microscopy Sciences) for 7 min., centrifuged at 600 rcf, washed with 1× PBS, and resuspended in 700 µL of 1× PBS for flow cytometry. Compensation was performed with unstained control cells, GFP+ Cnp-Mek1DD cells, and control cell samples individually stained with each antibody prior to all analyses with a BD LSRFortessa X-20 equipped with 5 lasers (355 nm, 405 nm, 488 nm, 561 nm, and 642 nm). 10000-50000 events were recorded for all analyses. Gating analysis was performed using FlowJo software.
Flow Sorting
After spleen dissociation as described above, splenic immune cells were resuspended at 20 x 10 6 cells/mL in FACS buffer. Immunostaining using anti-CD19-PE/Cy7 was performed at a concentration of 0.2 µg/mL in 2 mL total volume. After a 40 min. incubation in primary antibody, 1 mL of heat-inactivated FBS (F0926, Sigma) was added to each sample before centrifugation at 450 rcf for 6 min. Cells were counterstained with DAPI for 15 min. to exclude dead cells (1:10000). After centrifugation at 450 rcf for 6 min., cells were resuspended in 1 mL of FACS buffer for flow sorting. Live CD19 + B-cells were isolated for in vitro experiments using a BD FACSAria II SORP. In control samples the CD19 + population was collected, while in Cnp-Mek1DD samples the CD19 + GFP + population was collected. Cells were sorted into B-cell collection media containing 10% heat-inactivated FBS (F0926, Sigma), 2 mM l-glutamine, 50 µm 2-mercaptoethanol (21985023, Thermofisher Scientific), 1 mM sodium pyruvate (11360-070, Gibco), 10 mM HEPES (15630-080, Gibco), and 1× pen-strep in RPMI-1640.
B-Cell Culture
Flow-sorted CD19 + B-cells were centrifuged at 450 g then resuspended in 1 mL B-cell collection media (described above). Viable B-cells were counted using trypan blue to exclude dead cells. Samples were then centrifuged and resuspended at 2 x 10 6 /mL in B-cell culture media containing 10% heat-inactivated FBS (Lot# 15C487, F0926, Sigma), 2 mM l-glutamine, 50 µm 2-mercaptoethanol (21985023, Thermofisher Scientific), 1 mM sodium pyruvate (11360-070, Gibco), 1× pen-strep, and 10 µg/mL lipopolysaccharides (LPS; L2630, Sigma) in RPMI-1640. B-cells were plated in 96-well TSS plates at 2 x 10 5 /well (200 uL/well) and cultured at 37°C for 48 hrs. To examine the production of IL-10 specifically, additional stimulation was performed with PMA (50 ng/mL; P1585, Sigma) and ionomycin (500 ng/mL; I9657, Sigma) for the last 5 hrs. of culture ( Yanaba et al., 2008 ).
Quantitative Reverse Transcription Polymerase Chain Reaction
Stimulated B-cells were collected from individual wells on 96-well plates and centrifuged at 450 g for 5 min. Supernatant was removed and cells resuspended in 350 uL of RLT buffer from the Qiagen RNeasy Mini Prep kit (74104, Qiagen), then frozen at -80°C for a minimum of 24 hrs. before additional processing. After thawing on ice, samples were prepared with the QiaShredder columns (79656, Qiagen) for homogenization followed by isolation with the Qiagen RNA Mini Prep kit. RNA concentration and quality was quantified using the Nanodrop and 156 ng of RNA was used for each cDNA reaction using Superscript II (18064014, Invitrogen). For quantitative reverse transcription polymerase chain reaction (qRT-PCR), 1.5 uL of cDNA was used in each reaction with iTaq Universal SYBR Green Supermix (1725124, BioRad). Primers used were as follows: GAPDH Fwd: 5′- GATGCCCCCATGTTTGTGAT -3′, GAPDH Rev: 5’ GGTCATGAGCCCTTCCACAAT -3′, IL-10 Fwd: 5′- CAGCCGGGAAGACAATAACT -3′, IL-10 Rev: 5′- GTTGTCCAGCTGGTCCTTTG -3′.
Enzyme-Linked Immunosorbent Assay
Conditioned media was collected from stimulated B cells cultured on a 96-well plate and centrifuged for 5 min at 1500 rpm to remove debris. The media was incubated with the mouse IL-10 Quantikine ELISA kit (M1000B, R&D Systems) according to manufacturer’s protocol. Levels of IL-10 were detected using the Gene5 plate reader (BioTek) at an absorbance of 450 nm.
Statistical Analysis
For experiments comparing two groups, Student’s t test was used. When comparing two groups with multiple measurements, as for EAE scoring over time, two-way repeated measures ANOVA followed by Sidak’s multiple comparisons test was used. F and p statistics for 2-way repeated measures ANOVA are reported in relevant figure legends, and statistical significance in graphs is represented using p value results from Sidak’s multiple comparisons test. Sample sizes and p values are reported in each figure legend. All graphical data is represented as mean ± SEM. For all experiments, ****p ≤ 0.0001, ***p ≤ 0.001, **p ≤ 0.01, and *p ≤ 0.05 unless otherwise stated.
Cnp-Cre;Mek1DD/+ Mice Exhibit Improved EAE Clinical Score
Mice with sustained activation of ERK1/2 in the OL lineage exhibit hypermyelination throughout the CNS, and thicker remyelination in a focal LPC-induced demyelinated lesion of the spinal cord ( Fyffe-Maricich et al., 2013 ; Ishii et al., 2013 ). While previous studies used the LPC model of demyelination to examine loss and repair of myelin in the CNS, we wanted to determine whether sustained ERK1/2 activation in the OL lineage is beneficial in an immune-mediated demyelination model similar to MS. Therefore, we induced MOG 35-55 EAE in female Cnp-Mek1DD and control littermate mice at 10-14 weeks of age ( Figure 1A ). EAE clinical severity was assessed daily starting at 7 days post-induction (dpi) using the standard scoring protocol, with normal locomotion scored as 0 and moribund scored as 5. Cnp-Mek1DD mice exhibited a significantly reduced score versus control littermates from 13 dpi until the end of scoring at 24 dpi, nearly the entire disease course ( Figure 1B ; *p = 0.01, **p = 0.002 – 0.008, ***p = 0.0002 – 0.0005, ****p < 0.0001). Upon further examination, both peak score (Control = 3.7, Cnp-Mek1DD = 2.3; p = 0.004) and maximum improvement (Control = 0.4, Cnp-Mek1DD = 1.1; p = 0.02) were also significantly improved, while control animals exhibited a standard chronic MOG 35-55 disease course for C57Bl/6 mice ( Rangachari & Kuchroo, 2013 ; Terry et al., 2016 ). To validate the observed protective effect in Cnp-Mek1DD mice, we used tamoxifen inducible Plp-Cre ERT ;Mek1DD-eGFP/+ (Plp-Mek1DD) and CRE-negative control littermate mice, which exhibit recombination in PLP + mature OLs at the time of tamoxifen injection ( Ishii et al., 2016 ; Jeffries et al., 2016 ). In order to determine whether ERK1/2-induced hypermyelination was protective against the development or severity of ascending hind-limb paralysis caused by EAE-induced demyelination, we administered tamoxifen to 6-11 week old female Plp-Mek1DD and control littermate mice 40 days before inducing EAE ( Figure 1C ). Previous work has demonstrated that significant hypermyelination is observed in Plp-Mek1DD mice between 21-60 days post tamoxifen (dpt) ( Jeffries et al., 2016 ). EAE induction at 12-17 weeks of age resulted in comparable disease initiation, as measured by the timing of onset of clinical symptoms in both Plp-Mek1DD and control mice. Moreover, there were no significant differences in EAE clinical scores throughout the 28 days following EAE induction, suggesting that hypermyelination driven by ERK1/2 activation is not protective against EAE induction or the severity of the clinical signs that develop ( Figure 1D ; p = 0.71 – 0.99+). Cnp-Cre additionally causes loxP- mediated recombination in OPCs that give rise to newly-generated mature OLs responsible for remyelination. To address whether MEK1DD expression in OPCs results in improved functional score in the EAE model, we induced EAE in 10-12 week old female Pdgfrα-Cre ERT ;Mek1DD-eGFP/+ (Pdgfrα-Mek1DD) and control littermate mice. Upon exhibiting an EAE clinical score of 1 or greater, each mouse was given tamoxifen for five consecutive days to induce recombination in OPCs ( Figure 1E ). However, this did not result in any change in EAE disease course in Pdgfrα-Mek1DD mice compared to controls, suggesting that sustained ERK1/2 activity in OPCs does not improve functional score in EAE during remyelination ( Figure 1F ; p = 0.83 – 0.99+).
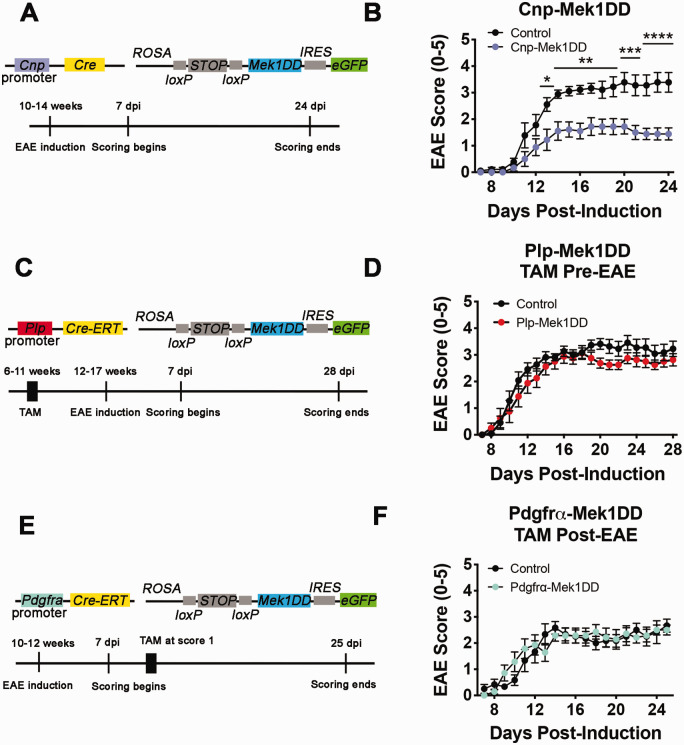
Cnp-Mek1DD Mice Display Reduced EAE Clinical Score Compared to Controls. A: Schematic diagram showing constructs for Cnp-Mek1DD mouse and the EAE induction paradigm used. B: EAE clinical scores from Cnp-Mek1DD (blue) and control (black) mice, n = 9/group. Two-way repeated measures ANOVA was significant (F(17,272) = 4.876; p < 0.0001). Significance depicted based on Sidak’s multiple comparisons test after two-way ANOVA. C: Schematic diagram showing constructs for Plp-Mek1DD mouse and the EAE induction paradigm used, with tamoxifen (TAM) injections prior to EAE induction. D: EAE clinical scores from control (black) and Plp-Mek1DD (red) mice given TAM prior to EAE induction, n = 8-11/group. Two way repeated measures ANOVA was not significant (F(21,357) = 0.75; p = 0.78). E: Schematic diagram showing constructs for Pdgfrα-Mek1DD mouse and the EAE induction paradigm used, with tamoxifen (TAM) injections given at score ≥ 1 for each mouse. F: EAE clinical scores from control (black) and Pdgfrα-Mek1DD (green) mice given TAM during EAE disease, n = 6-7/group. Two-way repeated measures ANOVA was not significant (F(18,198) = 1.21; p = 0.25). All graphical data are presented as mean ± SEM. *p ≤ 0.05, **p ≤ 0.01, ***p ≤ 0.001, ****p ≤ 0.0001.
Toluidine blue staining in spinal cord ventral white matter revealed that both Cnp-Mek1DD and Plp-Mek1DD mice displayed visible hypermyelination compared to controls at the end of EAE disease course comparable in magnitude to previously published observations ( Figure 2A and B ) ( Ishii et al., 2013 ; Jeffries et al., 2016 ). Luxol fast blue staining showed extensive lesions throughout the white matter of the control spinal cord at the end of EAE disease course, while no lesions were visible in the Cnp-Mek1DD spinal cord ( Figure 2C ). In order to further investigate changes in Cnp-Mek1DD EAE spinal cords compared to controls, we immunostained for myelin basic protein (MBP) at 24 dpi. MBP staining revealed widespread areas lacking MBP in control spinal cords. Areas with reduced MBP staining were also apparent in the white matter of the spinal cord of Cnp-Mek1DD mice, however, these were small and sparsely distributed ( Figure 2D ). We found that the percentage of white matter that lacked MBP expression was significantly decreased in the ventral white matter of Cnp-Mek1DD mice ( Figure 2D and E ; Control = 28.61%, Cnp-Mek1DD = 13.66%; p = 0.0006) but not in the dorsal white matter when quantifying MBP - area ( Figure 2D and E ; Control = 21.97%, Cnp-Mek1DD = 19.77%; p = 0.71). Despite this, we observed that the ventral white matter displayed unchanged IBA1 + area ( Figure 3A and B ; Control = 43.63%, Cnp-Mek1DD = 30.49%; p = 0.32), a marker for microglia and macrophages, while dorsal white matter IBA + was significantly decreased ( Figure 3A and B ; Control = 36.92%, Cnp-Mek1DD = 11.27%; p = 0.008). Finally, we immunostained control and Cnp-Mek1DD 24 dpi spinal cords for CD3, a marker of T-cells, and CD19, a marker of B-cells ( Figure 3C ). In all three control animals, extensive lesions, identified by DAPI hypercellularity, were observed throughout the ventral and dorsal white matter ( Figure 3C ). In contrast, Cnp-Mek1DD mice displayed very few, small lesions identified by DAPI, with one animal exhibiting no apparent lesions at all using this method. Within these lesions, we could identify both CD3 + T-cell infiltration and rare CD19 + B-cell infiltration ( Figure 3C ). These images highlight the very low lesion load of Cnp-Mek1DD mice during EAE despite apparent activation of microglia/macrophages and infiltration of CD3+ and CD19+ cells within existing lesions.
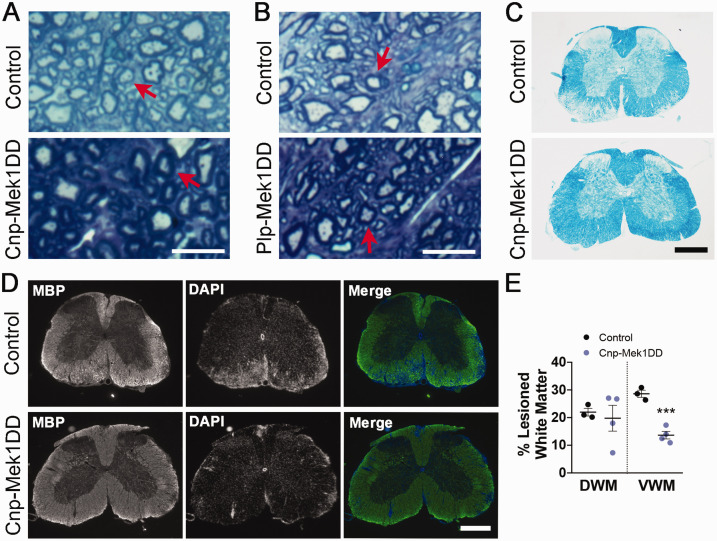
Cnp-Mek1DD Mice Display Hypermyelination and Reduced Lesion Load at the End of EAE Disease Course. A: Representative toluidine blue images from control and Cnp-Mek1DD spinal cord ventral white matter. Red arrows point to similarly sized axons. Scale bar = 10 µm. B: Representative toluidine blue images from control and Plp-Mek1DD spinal cord ventral white matter. Red arrows point to similarly sized axons. Scale bar = 10 µm. C: Luxol fast blue staining of control and Cnp-Mek1DD spinal cords at 24 dpi, scale bar = 500 µm. D: Myelin basic protein (MBP, green) and DAPI (blue) immunostaining of control and Cnp-Mek1DD spinal cords at 24 dpi, scale bar = 500 µm. E: The % of dorsal white matter (DWM) and ventral white matter (VWM) that is lesioned in control and Cnp-Mek1DD spinal cords, n = 3-4/group.
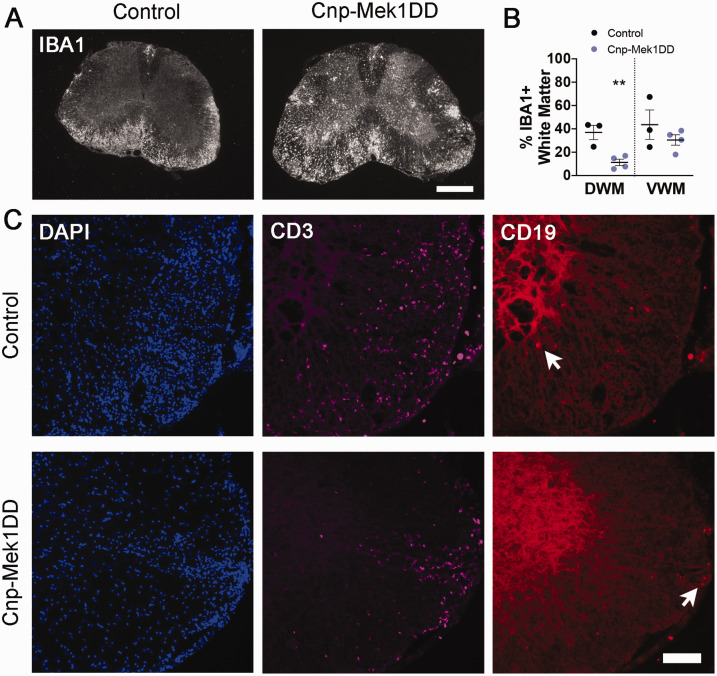
Cnp-Mek1DD Spinal Cords Exhibit Sparse Lesions With Evidence of Immune Cell Infiltration and Inflammation. A: IBA1 immunostaining of control and Cnp-Mek1DD spinal cords at 24 dpi, scale bar = 500 µm. B: The % of dorsal white matter (DWM) and ventral white matter (VWM) that is positive for IBA1 in control and Cnp-Mek1DD spinal cords, n = 3-4/group. C: Representative images of DAPI (blue), CD3 (magenta), and CD19 (red) immunostaining in control and Cnp-Mek1DD lesions at 24 dpi, scale bar = 100 µm. White arrows point to CD19+ B-cells.
While both Cnp-Mek1DD and Plp-Mek1DD mice displayed hypermyelination consistent with prior observations ( Fyffe-Maricich et al., 2013 ; Ishii et al., 2013 ; Jeffries et al., 2016 ), only Cnp-Mek1DD mice developed an attenuated clinical course of EAE disease. Additionally, data from Pdgfrα-Mek1DD mice demonstrated that MEK1DD expression in OPCs did not result in improved EAE disease course. Interestingly, while the loss of MBP was modest in the ventral white matter of Cnp-Mek1DD spinal cords compared to controls, an expansion of IBA1 + area was comparable to controls, and infiltration of CD3 + T-cells and CD19 + B-cells was occasionally observed.
The Cnp Promoter Drives CRE Expression in Splenic Immune Cell Populations
Our data in Cnp-Mek1DD mice revealed significant improvement in EAE clinical score compared to controls, yet data using tamoxifen-inducible Plp-Mek1DD or Pdgfrα-Mek1DD mice did not support the conclusion that MEK1DD expression in the OL lineage provides a protective or reparative functional benefit. These data were surprising, given that Cnp-Cre has previously been reported to cause recombination specifically in the OL lineage in the CNS ( Nishizawa et al., 1985 ; Trapp et al., 1988 ; Lappe-Siefke et al., 2003 ; Madsen et al., 2016 ; Mei et al., 2016 ; Joseph et al., 2019 ; Y. Yue et al., 2019 ). However, there are reports of Cnp promoter activity in cell types outside of the CNS, including immune cell populations ( Sheedlo et al., 1984 ; McFerran & Burgoyne, 1997 ; Miyoshi et al., 2001 ; Davidoff et al., 2002 ; F. Yue et al., 2014 ). Therefore, we hypothesized that Cnp-Cre was driving recombination to enable expression of MEK1DD in immune cells that mediate EAE. In order to determine whether this was the case, we examined immune cell populations from the spleens of 8-11 week old female Cnp-Mek1DD and control littermate mice. To our surprise, we discovered that ∼25-40% of live splenic cells were GFP + (data not shown) in Cnp-Mek1DD mice, while CRE-negative controls only exhibited 1-3% GFP + background. The absence of CRE but presence of the R26Stop Fl Mek1DD-eGFP transgene in controls supports the conclusion that the GFP + population is a result of CRE-mediated recombination of the transgene. Upon further gating analysis, we determined that ∼50% of CD19 + B-cells co-expressed GFP ( Figure 4A and E ; Control = 0.92%, Cnp-Mek1DD = 52.61%), ∼30% of CD11b + monocytes co-expressed GFP ( Figure 4B and E ; Control = 7.58%, Cnp-Mek1DD = 29.18%), ∼25% of CD3 + CD4 + T-cells co-expressed GFP ( Figure 4C and E ; Control = 1.20%, Cnp-Mek1DD = 24.56%), and ∼30% of CD3 + CD8 + T-cells co-expressed GFP ( Figure 4D and E ; Control = 2.45%, Cnp-Mek1DD = 30.49%). In B-cell and T-cell populations, we observed GFP hi and GFP lo expressing clusters that were both identified as GFP + for analysis. Due to variability in the position of the GFP lo cluster in the CD19 + B-cell population, in some samples the GFP lo group could not be included in the gate, resulting in two distinct trials exhibiting ∼40% or ∼70% recombination ( Figure 4E ). However, based on the presence of GFP hi and GFP lo CD19 + B-cells in all samples regardless of gating, we suggest that Cnp-Cre drives loxP recombination in ∼70% of CD19 + B-cells rather than ∼50%. In addition, we examined GFP + splenic cells and found that ∼80% of the GFP + cells were CD19 + B-cells while ∼20% of the GFP+ cells were CD3 + T-cells and only ∼4% were CD11b+ monocytes revealing that in the spleen, Cnp-Cre primarily drives loxP recombination in B-cells and T-cells ( Figure 4F and G ).
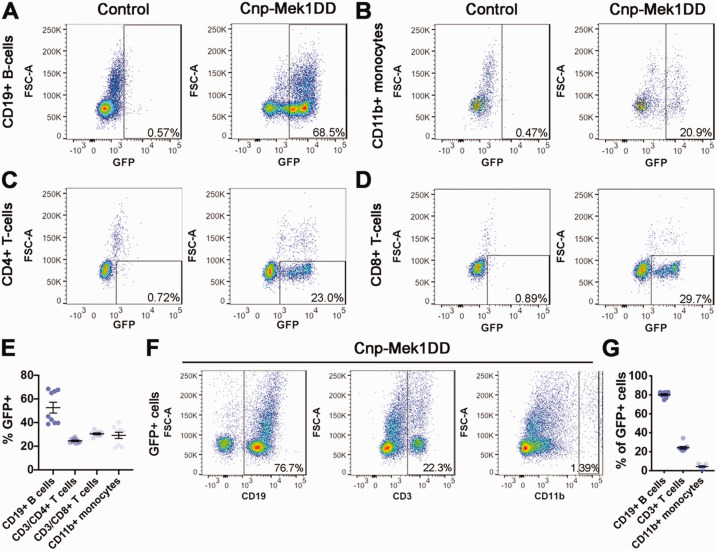
Cnp-Mek1DD Mice Display GFP+ Recombined Splenic B-Cells, Monocytes, and T-cells. A: Representative flow cytometry dot plots from control and Cnp-Mek1DD splenic cells showing CD19 + B-cells that are GFP+, n = 6-9/group. B: Representative flow cytometry dot plots from control and Cnp-Mek1DD splenic cells showing CD11b + monocytes that are GFP+, n = 6-9/group. C: Representative flow cytometry dot plots from control and Cnp-Mek1DD splenic cells showing CD3 + CD4 + T-cells that are GFP+, n = 6-9/group. D: Representative flow cytometry dot plots from control and Cnp-Mek1DD splenic cells showing CD3 + CD8 + T-cells that are GFP+ n = 6-9/group. E: Graph of the % of CD19 + , CD3 + CD4 + , CD3 + CD8 + , and CD11b + cells that are GFP+ in Cnp-Mek1DD spleens, n = 9. F: Representative flow cytometry dot plots from Cnp-Mek1DD splenic cells showing GFP+ cells that are CD19 + , CD11b + , or CD3 + , n = 9. G: Graph of the % of GFP+ cells that are CD19 + , CD3 + , or CD11b + in Cnp-Mek1DD spleens, n = 9.
We next examined recombination using female Cnp-Cre;ROSA mT/mG (Cnp-DR) carrying a double reporter that results in expression of membrane-bound GFP in recombined cells. This enabled us to evaluate Cnp-Cre mediated recombination without the presence of the R26Stop Fl Mek1DD-eGFP transgene, which might shift splenic immune cell populations. Flow analysis for GFP, CD45, CD19, CD3, and CD11b revealed that nearly 90% of CD19 + B-cells (Supplemental Figure 1A; Control = 0.81%, Cnp-DR = 88.43%), ∼40% of CD11b + monocytes (Supplemental Figure 1B; Control = 1.40%, Cnp-DR = 42.80%), and ∼50% of CD3 + T-cells (Supplemental Figure 1C; Control = 0.55%, Cnp-DR = 49.10%) were recombined. The proportionally higher recombination rates may suggest that the stronger membrane-bound GFP reporter expressed by Cnp-DR mice identifies additional recombined cells that the fainter cytoplasmic GFP reporter expressed by Cnp-Mek1DD mice does not. Importantly, these data further demonstrate that Cnp-Cre drives recombination in splenic immune cell populations, particularly in CD19 + B-cells.
In order to confirm that CRE-mediated recombination in splenic immune populations occurs under control of the Cnp promoter and not the Plp promoter, we conducted flow cytometry for GFP, CD45, CD19, CD3, and CD11b on Plp-DR mice. Female Plp-DR and control littermate mice without Plp-Cre ERT were administered tamoxifen to induce recombination at 11 weeks of age, and spleens collected 2 weeks after the first tamoxifen injection for analysis. Importantly, we did not observe any GFP+ populations in the spleen when gating for CD19 + B-cells, CD11b + monocytes, or CD3 + T-cells ( Figure 5A to C ). The absence of GFP+ splenic immune populations in Plp-DR mice supports our conclusion that the Cnp promoter drives the expression of CRE resulting in recombination in splenic immune cell populations, while other promoters that drive expression in the OL-lineage such as Plp do not. Taken together, these data suggest that in addition to the OL lineage, the Cnp promoter drives CRE expression in splenic B-cells, T-cells, and monocytes, immune cell populations known to regulate EAE and other autoimmune diseases.
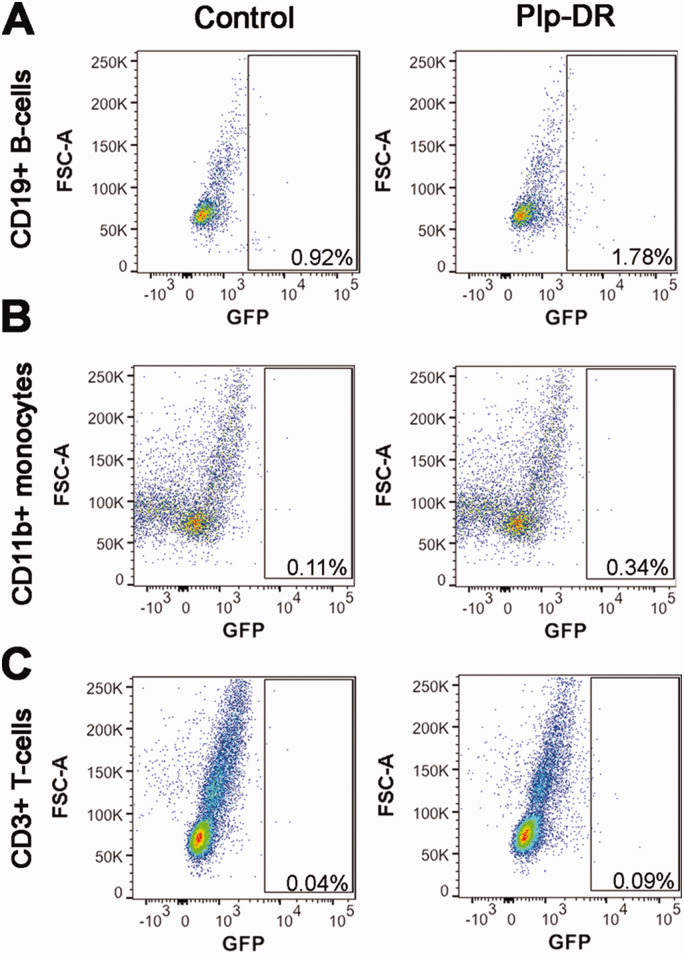
Plp-Cre ERT Does Not Drive Recombination in Splenic Immune Cells. A: Representative flow cytometry dot plots from control and Plp-DR splenic cells showing CD19 + B-cells that are GFP+, n = 3/group. B: Representative flow cytometry dot plots from control and Plp-DR splenic cells showing CD11b + monocytes that are GFP+, n = 3/group. C: Representative flow cytometry dot plots from control and Plp-DR splenic cells showing CD3 + T-cells that are GFP+, n = 3/group.
Constitutively Active MEK1 Expression Increases In Vitro Activation of Splenic CD19 + B-Cells
MOG 35-55 EAE was originally thought to be a T-cell mediated disease independent of B-cell function ( Mendel et al., 1995 ; Lyons et al., 1999 ; Jager et al., 2009 ). However, recent studies and the clinical success of B-cell targeted drugs such as rituximab have revealed important roles for B-cell populations in EAE and MS ( Mann et al., 2012 ; Pierson et al., 2014 ; Ray & Basu, 2014 ; Parker Harp et al., 2015 ; Hausser-Kinzel & Weber, 2019 ; Pennati et al., 2020 ). While GFP + recombined B-cells, T-cells, and monocytes were observed, recombination was higher in CD19 + B-cells than T-cells or monocytes ( Figure 2A to E ), and the majority of GFP + cells were CD19 + ( Figure 2F and G ). Additionally, limited research has been done to determine the role of ERK1/2 signaling in the function of specific B-cell populations. We first wanted to determine whether expression of MEK1DD resulted in baseline splenic B-cell population changes. In order to address this, we labeled splenic cells for CD19, CD1d, CD5, and CD138, each of which mark a defined subset of B-cells when combined with the CD19 pan-B-cell marker. The CD19 + CD5 + CD1d hi B-cell population is associated with a regulatory B10 cell subset, which has previously been shown to suppress EAE disease severity ( Matsushita et al., 2008 ; 2010 ). Conversely, the CD19 + CD138 + population marks plasmablasts, which eventually differentiate into antibody-producing CD19 - CD138 + plasma cells that can positively or negatively modulate EAE inflammation ( Shen et al., 2014 ; Chen et al., 2016 ; Pollok et al., 2017 ; Fillatreau, 2019 ). We did not observe any significant differences in the percentages of CD19 + B-cells in the spleen ( Figure 6A and D ; Control = 36.60%, Cnp-Mek1DD = 40.88%; p = 0.54), CD5 + CD1d hi cells out of CD19 + B-cells ( Figure 6B and E ; Control = 2.22%, Cnp-Mek1DD = 3.03%; p = 0.12), or CD138 + cells out of CD19 + B-cells ( Figure 6C and E ; Control = 9.86%, Cnp-Mek1DD = 12.2%; p = 0.07). However, it was of particular interest for us to examine how the expression of MEK1DD in CD19 + splenic B-cells affects activation, which could in turn influence EAE disease course. We labeled splenic cells from Cnp-Mek1DD and control mice for CD19, then flow sorted for either CD19 + cells (in control) or CD19 + GFP + cells (in Cnp-Mek1DD). Importantly, flow sorting confirmed a recombination rate of ∼70% in CD19 + B-cells, with 7 Cnp-Mek1DD samples exhibiting GFP positivity between 62% and 78.2%, with a mean of 72.3% (data not shown). CD19 + cells from control and CD19 + GFP + cells from Cnp-Mek1DD spleens were then cultured for 72 hrs. and stimulated with LPS in order to induce B-cell proliferation and activation. After 72 hrs. in culture, B-cells were labeled for CD19, MHC-II, CD5, CD1d, and CD138 and analyzed by flow cytometry. Flow analysis revealed that LPS activation resulted in successful activation of control CD19 + B-cells, with a high-expressing MHC-II + group evident in both control and Cnp-Mek1DD samples ( Figure 7A and D ). The percentage of CD19 + B-cells that labeled as MHC-II hi was unchanged between controls and Cnp-Mek1DD stimulated B-cells ( Figure 7A and D ; Control = 32.75%, Cnp-Mek1DD = 46.53%; p = 0.28). However, when examining the percentage of CD5 + CD1d hi cells within the CD19 + population, we observed a 5-fold significant increase in the numbers of Cnp-Mek1DD B-cells within this population compared to controls, a subset of which are regulatory B10 cells ( Figure 7B and E ; Control = 2.33%, Cnp-Mek1DD = 10.82%; p = 0.008). Additionally, we observed a 3-fold significant increase in the percentage of CD19 + B-cells expressing CD138 compared to controls, suggesting increased differentiation of B-cells into plasmablasts ( Figure 7C and E ; Control = 7.22%, Cnp-Mek1DD = 19.87%; p = 0.003). Taken together, these data suggest that activated ERK1/2 in CD19 + B-cells results in increased expansion of CD5 + CD1d hi and CD138 + B-cell subsets, both of which modulate EAE disease severity ( Matsushita et al., 2008 , 2010 ; Shen et al., 2014 ; Fillatreau, 2019 ).
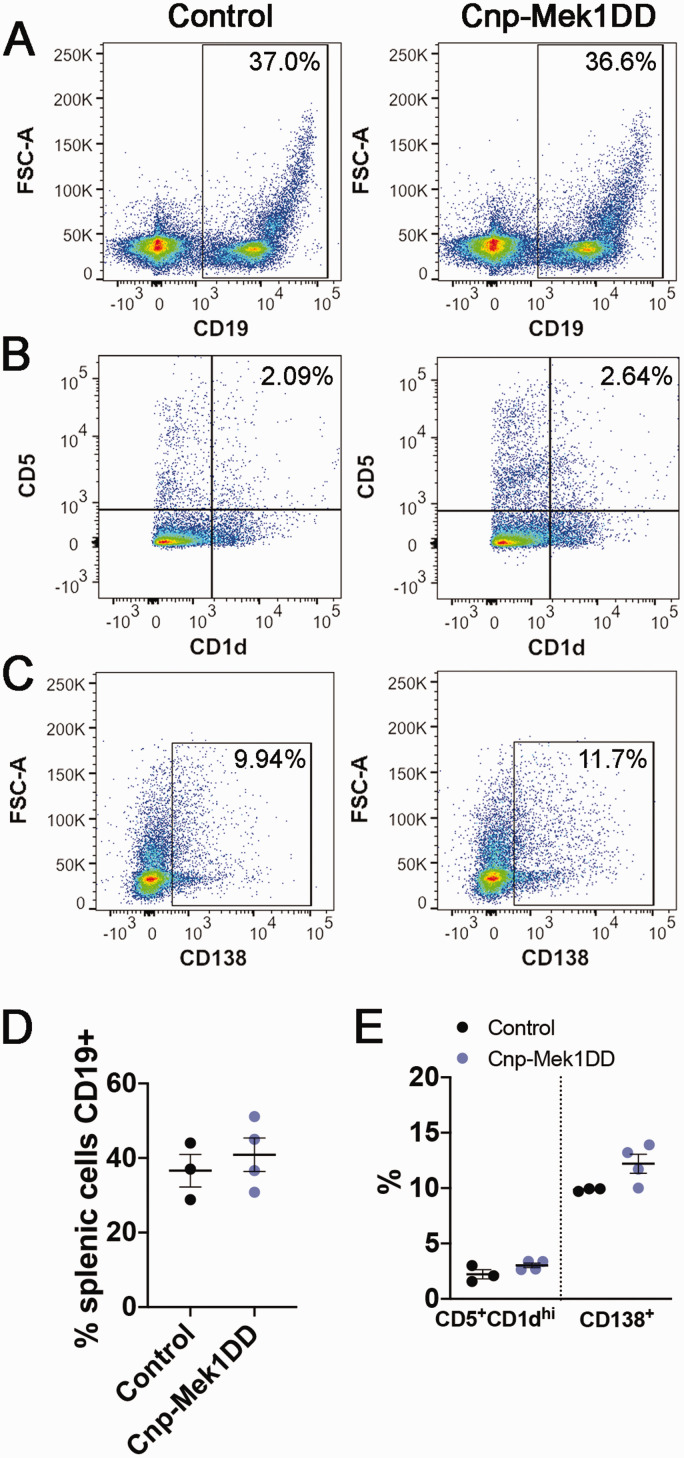
MEK1DD Expression in Splenic B-Cells Does Not Alter B-Cell Populations Pre-Stimulation. A: Representative flow cytometry dot plots from control and Cnp-Mek1DD splenic cells showing CD19 + B-cells, n = 3-4/group. B: Representative flow cytometry dot plots from control and Cnp-Mek1DD splenic cells showing CD5 + CD1d hi B-cells, n = 3-4/group. C: Representative flow cytometry dot plots from control and Cnp-Mek1DD splenic cells showing CD138 + plasmablasts, n = 3-4/group. D: Graph showing % of control or Cnp-Mek1DD splenic cells expressing CD19, n = 3-4/group. E: Graph showing % of CD19 + control or Cnp-Mek1DD splenic cells that are CD5 + CD1d hi or CD138 + , n = 3-4/group.
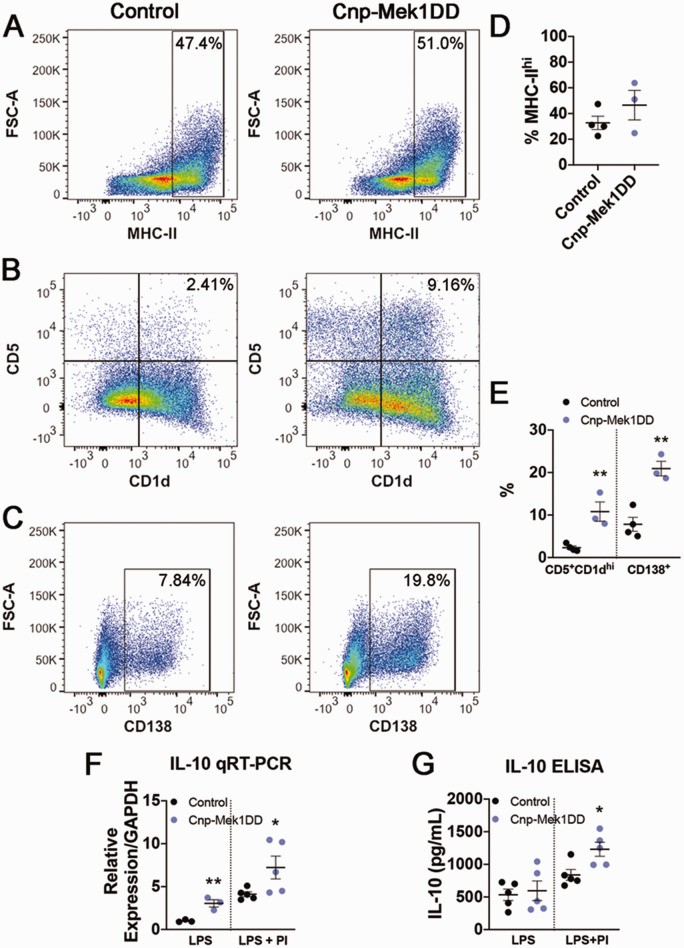
MEK1DD Expression Results in Increased B-Cell Activation After In Vitro LPS Stimulation. A: Representative flow cytometry dot plots from control and GFP+ LPS-stimulated B-cells showing MHC-II hi B-cells, n = 3-4/group. B: Representative flow cytometry dot plots from control and GFP+ LPS-stimulated B-cells showing CD5 + CD1d hi B-cells, n = 3-4/group. C: Representative flow cytometry dot plots from control and GFP+ LPS stimulated B-cells showing CD138 + plasmablasts, n = 3-4/group. D: Graph of the % of CD19 + cells that are high-expressing MHC-II in control and Cnp-Mek1DD GFP+ LPS-stimulated B-cells, n = 3-4/group. E: Graph of the % of CD19 + B-cells that are CD5 + CD1d hi or CD138 + in control and Cnp-Mek1DD GFP+ LPS stimulated B-cells, n = 3-4/group. F: Relative mRNA expression of IL-10 in LPS-stimulated or LPS+PI-stimulated control and Cnp-Mek1DD GFP+ B-cells, n = 3-5/group. *p ≤ 0.05, **p ≤ 0.01.
MEK1DD Expression in Splenic CD19+ B-Cells Results in Upregulated IL-10 Expression
Cytokine release by immune cells significantly impacts the severity and progression of EAE disease. For example, the proinflammatory cytokine IL-6 is critical for the induction of EAE through the modulation of Th1, Th2, and Th17 cell activation and function ( Samoilova et al., 1998 ; Okuda et al., 1999 ; Serada et al., 2008 ), while the anti-inflammatory cytokine IL-10 significantly reduces the severity of EAE disease ( Bettelli et al., 1998 ; Xiao et al., 1998 ; Cua et al., 1999 ). Specifically, IL-10 production by regulatory B10 cells can suppress EAE disease initiation in a manner similar to clinical scores seen in Cnp-Mek1DD mice ( Matsushita et al., 2010 ). We wanted to determine whether the significant increase in the proportion of CD5 + CD1d hi cells in the Cnp-Mek1DD mice correlated with a significant increase in the expression of IL-10, which is the cytokine that B10 cells must produce in order to elicit an immunosuppressive effect in EAE. Therefore, we ran qRT-PCR for IL-10 using RNA isolated from LPS-stimulated control and Cnp-Mek1DD B-cells. We observed a 3-fold significant increase in IL-10 transcription from Cnp-Mek1DD LPS-stimulated B-cells compared to controls ( Figure 7F ; normalized to control, Cnp-Mek1DD = 3.05; p = 0.009). While published data indicate that LPS stimulation results in the expansion of IL-10 producing B10 cells, IL-10 production by these cells is further increased with the addition of phorbol 12-myristate 13-acetate (PMA) and ionomycin in the last 5 hrs. of culture ( Yanaba et al., 2008 ). Based on this, we also isolated RNA from LPS and PMA+ionomycin (PI)-stimulated control and Cnp-Mek1DD B-cells. qRT-PCR analysis revealed that while control B-cells upregulated IL-10 transcription, this increase in expression was significantly higher in Cnp-Mek1DD B-cells ( Figure 7F ; normalized to LPS-stimulated control, Control = 4.12, Cnp-Mek1DD = 7.23; p = 0.05). We then used an ELISA assay to determine whether B-cells from Cnp-Mek1DD mice secreted more IL-10 into the culture media over 48 hours in response to stimulation. While LPS stimulation alone did not result in increased IL-10 secretion ( Figure 7G ; Control = 533.4 pg/mL, Cnp-Mek1DD = 595 pg/mL; p = 0.73), media from LPS+PI-stimulated Cnp-Mek1DD B-cells exhibited a significant increase in IL-10 levels compared to control media ( Figure 7G ; Control = 838.3 pg/mL, Cnp-Mek1DD = 1231 pg/mL; p = 0.02). Taken together, these data support the conclusion that the increased response to LPS stimulation by Cnp-Mek1DD B-cells results in enhanced expansion of IL-10 producing B-cell subsets, such as B10 cells, and upregulation of anti-inflammatory cytokine IL-10 transcription and secretion after LPS+PI stimulation.
The ERK1/2 signaling pathway was previously implicated in regulating remyelination efficiency and thickness, with mice lacking ERK2 in the OL lineage displaying delayed remyelination and mice expressing MEK1DD in the OL lineage exhibiting accelerated remyelination with thicker myelin sheaths after LPC demyelination ( Fyffe-Maricich et al., 2013 ; Michel et al., 2015 ). With the impressive results from these LPC studies in Cnp-Mek1DD mice, we wanted to examine how MEK1DD expression in the OL lineage might affect immune-mediated de- and remyelination. Strikingly, we observed a significant improvement in EAE disease course and white matter lesion load in Cnp-Mek1DD mice compared to controls. However, we were surprised when experiments using Plp-Mek1DD mice indicated that ERK1/2 activation in existing OLs during demyelination did not also protect against EAE induction. Instead, our data suggest an important role for ERK1/2 signaling in immune cells, particularly CD19 + splenic B-cells, which may modulate EAE severity.
Our data showing no difference in clinical EAE score severity in Plp-Mek1DD mice given tamoxifen prior to EAE induction compared to controls suggests that the functional protection exhibited by Cnp-Mek1DD mice is not due to hypermyelination. It is important to note that our data, in line with previous studies, indicates the extent of hypermyelination is greater in Cnp-Mek1DD mice compared to Plp-Mek1DD mice ( Fyffe-Maricich et al., 2013 ; Ishii et al., 2013 ; 2016 ; Jeffries et al., 2016 ). Although we initially hypothesized that hypermyelination would be protective against EAE, existing data suggest that extreme hypermyelination similar to that seen in Cnp-Mek1DD mice can exacerbate EAE severity ( Jaini et al., 2013 ). Therefore, while we cannot rule out the possibility that hypermyelination in Cnp-Mek1DD mice could synergistically provide protection against EAE, it is likely that immune modulation is the chief factor in the observed suppression of EAE clinical scores.
Pdgfrα-Mek1DD mice given tamoxifen to induce MEK1DD expression in OPCs during EAE had similar disease course to controls, suggesting that enhanced ERK signaling in adult OPCs, and the population of remyelinating OLs that they give rise to, is not sufficient to lead to functional improvements in the context of immune-mediated demyelination . Additionally, Plp-Mek1DD mice did not exhibit functional improvement despite existing evidence that pre-existing mature OLs expressing MEK1DD can contribute to remyelination after LPC demyelination ( Jeffries et al., 2016 ). Conversely, Cnp-Mek1DD mice showed significantly better functional improvement in EAE score compared to controls, along with the initial reduction in EAE severity. It is possible that the inflammatory environment of EAE and ongoing demyelination may functionally override any improvement on remyelination thickness, such that even if Pdgfrα-Mek1DD or Plp-Mek1DD mice do exhibit thicker remyelination similar to that observed in Cnp-Mek1DD mice after LPC injection ( Fyffe-Maricich et al., 2013 ), it is not sufficient to alter clinical EAE score. This is possible since it has been shown that inflammation and axonal pathology contribute heavily to functional impairment in EAE ( Wujek et al., 2002 ; Lassmann & Bradl, 2017 ). We further cannot eliminate the possibility that it is a combination of improved remyelination and altered immunological response that results in the improved EAE phenotype of Cnp-Mek1DD mice. Experiments dissecting the potential contributions of immune cell modulation and improved remyelination to either the observed suppression of EAE disease progression or the improvement of EAE functional score will be of importance in future studies.
Cnp-Cre mice until now have been used as an OL lineage and Schwann cell-specific Cre mouse line. However, there have been previous reports of Cnp gene expression in cells outside the CNS, which the original study using Cnp-Cre mice mentioned in brief ( Lappe-Siefke et al., 2003 ). Mouse ENCODE Consortium transcriptome data reveals high levels of Cnp transcription in adult spleen and thymus along with brain tissues ( F. Yue et al., 2014 ). Additionally, a study using Northern blotting to examine Cnp1 transcription in mouse tissues showed that while expression is highest in the brain, Cnp1 expression is also evident in spleen, heart, liver, and lung ( Miyoshi et al., 2001 ). Another study demonstrated that CNPase activity appears to be highest outside of the CNS in the spleen and thymus ( Weissbarth et al., 1981 ). More specifically, a study examining CNPase enzymatic activity revealed that while myelin indeed displays the highest level of CNPase activity, both human and rat lymphocytes exhibit measurable CNPase activity ( Sheedlo et al., 1984 ). Finally, human lymphocytes showed increased CNPase activity in vitro when stimulated with a mitogenic lectin from Robinia pseudoacacia , although curiously, the researchers did not observe a significant increase in CNPase activity when stimulating fractionated T-cells or B-cells alone ( Sabeur et al., 1986 ). These studies strongly support our conclusion that the Cnp promoter is active and drives CRE expression in splenic immune cells. While previously undescribed in studies using the Cnp-Cre mouse, it will be of critical importance to consider immune cell function when using Cnp-Cre mice in future research.
Despite the knowledge that CNPase is expressed and active in splenic tissues and immune cells, EAE studies using mice with gene expression or deletion driven by the Cnp promoter have variably addressed this. When pancreatic ER kinase (PERK) is deleted using Cnp-Cre, this results in detectable loxP recombination in tail, heart, and lung tissue in addition to the brain, but not in spleen or thymus, in contrast to our data using GFP expression ( Hussien et al., 2014 ). While these mice display worsened EAE, no change in T-cell infiltration or macrophage/microglial activation was observed. Similarly, PCR analysis of activating transcription factor 4 (ATF4) deletion using Cnp-Cre in a second publication did not reveal loxP recombination in any tissue outside of the CNS, and these mice displayed comparable EAE disease to controls (Y. Yue et al., 2019 ). A third publication demonstrated striking attenuation of EAE severity in adult Cnp-Cre;Chrm1 fl/fl mice without effect on developmental myelination, but potential effects on immune function were not addressed ( Mei et al., 2016 ). Another study used Cnp-Cre to delete tumor necrosis factor receptor 2 (TNFR2), resulting in exacerbated EAE disease course ( Madsen et al., 2016 ). Interestingly, in this report the researchers observed increased T-cell infiltration during peak disease, but did not investigate whether recombination occurred in immune cell populations. In a publication using Cnp-Cre to express ovalbumin (OVA) neoepitopes, adoptive transfer of OVA-specific T-cells worsened EAE severity; these mice displayed increased T-cell infiltration and myeloid cell numbers but no analysis of potential recombination in host immune cell populations was performed ( Rayasam et al., 2018 ). One study used the Cnp expression cassette, rather than Cnp-Cre, to express dominant negative interferon regulatory factor 1 (IRF-1), resulting in strongly attenuated EAE disease course ( Ren et al., 2011 ). Importantly, this study validated CNS-specific expression of the dominant negative IRF-1 downstream of the Cnp expression cassette, and no changes in peripheral immune cell populations or function were observed. The variable data on immune cell recombination when using the Cnp promoter in EAE studies further underscore the importance of considering potential changes to immune function in these models.
Our results indicate that Cnp-Mek1DD spleens exhibit approximately 70% CD19 + B-cell recombination, 30% CD11b + monocyte recombination, 25% CD4 + T-cell recombination, and 30% CD8 + T-cell recombination. However, Plp-DR mice did not demonstrate splenic recombination, suggesting that Plp-Mek1DD mice, unlike Cnp-Mek1DD mice, do not have MEK1DD-expressing splenic immune cells during EAE that may be contributing to suppression of EAE disease initiation. Cnp-Mek1DD mice revealed reduced EAE clinical severity versus controls while Plp-Mek1DD EAE scores were comparable to controls. Therefore, the decrease in severity of EAE clinical scores observed in Cnp-Mek1DD mice is likely being driven primarily by altered immune cell function and not enhanced ERK1/2 signaling in the OL-lineage. Previous literature has revealed ERK1/2 signaling promotes the activation of CD4 + T-cells thought to be primarily responsible for EAE induction, while also driving activation of cytotoxic CD8 + T-cells and suppressing and destabilizing CD4 + FoxP3 + regulatory T-cells ( Dumont et al., 1998 ; D’Souza et al., 2008 ; Luo et al., 2008 ; Chang et al., 2012 ; Liu et al., 2013 ; Guo et al., 2014 ). In particular, ERK1/2 signaling has been shown in several studies to activate EAE-inducing Th1 and Th17 cells ( Chang et al., 2012 ; Liu et al., 2013 ). In addition, ERK1/2 signaling has been widely studied in macrophage development and activation, with evidence for ERK1/2 activation promoting proinflammatory polarization ( Rao, 2001 ; Traves et al., 2012 ). Because the expression of MEK1DD results in sustained ERK1/2 activation, it seems unlikely that recombined monocyte or T-cell populations in Cnp-Mek1DD mice are the cause of reduced EAE severity. However, it is important to note that we cannot eliminate the possibility that effects on these cell populations contribute to the observed effect on EAE disease course. Therefore, future studies will need to examine the role of ERK1/2 signaling in Cnp-Mek1DD T-cells and macrophages during EAE.
With the recent clinical success of B-cell targeted drugs like Ocrevus and Rituximab, there has been increasing interest in the roles of various B-cell populations in MS. Studies using the EAE model of demyelination have revealed mixed results in regard to B-cell necessity and function. While some reports have suggested that B-cells are unnecessary for EAE induction and progression, others have shown important modulatory roles for B-cells in EAE disease ( Mann et al., 2012 ; Pierson et al., 2014 ; Ray & Basu, 2014 ; Parker Harp et al., 2015 ; Hausser-Kinzel & Weber, 2019 ). In addition to this, previous work has highlighted the importance of ERK1/2 signaling in B-cell development and activation, with B-cell Receptor (BCR) ligation resulting in ERK1/2 activation ( Richards et al., 2001 ; Jacob et al., 2002 ; Rui et al., 2006 ; Gold, 2008 ; Teodorovic et al., 2014 ; Adem et al., 2015 ). When stimulated in vitro, B-cells expressing MEK1DD exhibited increased numbers of CD5 + CD1d hi B-cells as well as CD138 + plasmablasts, along with significantly upregulated IL-10 transcription and secretion. Previous research has found that both regulatory B10 cells, associated with the CD5 + CD1d hi subset, as well as CD138 + plasmablasts are able to secrete IL-10 ( Matsushita et al., 2008 , 2010 ; Matsumoto et al., 2014 ; Shen et al., 2014 ; Rojas et al., 2019 ). Multiple studies have shown the importance of IL-10 in modulating EAE severity ( Bettelli et al., 1998 ; Xiao et al., 1998 ; Cua et al., 1999 ). In particular, increased IL-10 expression by regulatory B10 cells has been shown to inhibit the development of EAE disease in a pattern similar to that observed in Cnp-Mek1DD mice, despite low B-cell infiltration into the CNS ( Matsushita et al., 2010 ; Mann et al., 2012 ). Excitingly, very recent publications demonstrate that the adoptive transfer of IL-10 producing regulatory B10 cells results in immunosuppression and concurrently promotes oligodendrogenesis and remyelination in the EAE model ( Pennati et al., 2016 ; 2020 ). ERK1/2 signaling has been implicated in IL-10 expression in multiple cell types ( Saraiva & O’Garra, 2010 ; Iyer & Cheng, 2012 ; Kubo & Motomura, 2012 ; Sanin et al., 2015 ; Garaud et al., 2018 ; Zhang & Kuchroo, 2019 ). Of particular interest are data revealing that in both human and mouse B-cells, TLR-mediated ERK1/2 activation is critical to induce IL-10 production, suggesting that the expression of MEK1DD may directly upregulate IL-10 transcription in splenic B-cells ( Liu et al., 2014 ; Sutavani et al., 2018 ). Taken together, these data suggest that ERK1/2 signaling promotes splenic B-cell activation and the expansion of CD5 + CD1d hi and CD138 + B-cell subsets, and may drive IL-10 expression in one or both of these populations.
This study presents novel data indicating that Cnp-Cre drives recombination in splenic immune cell populations, in particular CD19 + B-cells. Additionally, we determined that sustained ERK1/2 signaling in splenic B-cells results in enhanced activation of CD5 + CD1d hi and CD138 + subsets of B-cells and increased IL-10 expression, which may contribute to the inhibition of MOG 35-55 EAE disease progression. In conclusion, our data support the concept that downstream effectors of the ERK1/2 signaling pathway modulating B-cell function may be potential therapeutic targets in immune-mediated demyelinating diseases such as MS.
Summary Statement
ERK1/2 activation solely in oligodendrocyte-lineage cells does not provide a functional benefit in the context of EAE-mediated demyelination. Sustained ERK1/2 activation in both OL-lineage as well as B-cells, however, results in increased IL-10 expression and the attenuation of immune-mediated demyelination.
Acknowledgments
The authors would like to thank Dr. Wendy Macklin, Luipa Khandker, and Angelina Evangelou for helpful discussions and comments on this manuscript. We would like to thank Mara Sullivan and the Center for Biologic Imaging at the University of Pittsburgh for the thick toluidine blue sections. We also thank Tammy Mui-Galenkamp and the NJMS Flow Cytometry and Immunology Core Laboratory for the flow sorting to isolate CD19 + B-cells, as well as Dr. Sukhwinder Singh for his valuable expertise with flow cytometry.
Author Contributions: M. A. J., S. L. F.-M., and T. L. W. designed research; M. A. J., A. E. O., and K. U. performed research; M. A. J., A. E. O., and K. U. analyzed data; M. A. J., A. E. O., K. U., S. L. F.-M., and T. L. W. wrote the paper.
Declaration of Conflicting Interests: The author(s) declared no potential conflicts of interest with respect to the research, authorship, and/or publication of this article.
Funding: The author(s) disclosed receipt of the following financial support for the research, authorship, and/or publication of this article: This work was supported by an NMSS-Career Transition Fellowship to S. L. F.-M., NIH R01 NS091084 to S. L. F.-M., NIH R01 NS082203 to T. L. W., NMSS RG170728557 to T. L. W., and NIH F31 NS108521 to M. A. J.
ORCID iDs: Marisa A. Jeffries https://orcid.org/0000-0002-2486-0457
Alison E. Obr https://orcid.org/0000-0003-0949-3131
Teresa L. Wood https://orcid.org/0000-0002-4480-660X
Supplemental Material: Supplementary material for this article is available online.
- Adem J., Hamalainen A., Ropponen A., Eeva J., Eray M., Nuutinen U., Pelkonen J. (2015). ERK1/2 has an essential role in B cell receptor- and CD40-induced signaling in an in vitro model of germinal center B cell selection. Molecular Immunology, 67(2 Pt B), 240–247. [ DOI ] [ PubMed ] [ Google Scholar ]
- Bettelli E., Das M. P., Howard E. D., Weiner H. L., Sobel R. A., Kuchroo V. K. (1998). IL-10 is critical in the regulation of autoimmune encephalomyelitis as demonstrated by studies of IL-10- and IL-4-deficient and transgenic mice. Journal of Immunology (Baltimore, MD: 1950), 161(7), 3299–3306. [ PubMed ] [ Google Scholar ]
- Chang C. F., D'Souza W. N., Ch'en I. L., Pages G., Pouyssegur J., Hedrick S. M. (2012). Polar opposites: Erk direction of CD4 T cell subsets . Journal of Immunology (Baltimore, MD: 1950), 189(2), 721–731. [ DOI ] [ PMC free article ] [ PubMed ] [ Google Scholar ]
- Chen D., Ireland S. J., Davis L. S., Kong X., Stowe A. M., Wang Y., White W. I., Herbst R., Monson N. L. (2016). Autoreactive CD19+CD20- Plasma cells contribute to disease severity of experimental autoimmune encephalomyelitis. Journal of Immunology (Baltimore, MD: 1950), 196(4), 1541–1549. [ DOI ] [ PubMed ] [ Google Scholar ]
- Cua D. J., Groux H., Hinton D. R., Stohlman S. A., Coffman R. L. (1999). Transgenic interleukin 10 prevents induction of experimental autoimmune encephalomyelitis. The Journal of Experimental Medicine, 189(6), 1005–1010. [ DOI ] [ PMC free article ] [ PubMed ] [ Google Scholar ]
- Davidoff M. S., Middendorff R., Kofuncu E., Muller D., Jezek D., Holstein A. F. (2002). Leydig cells of the human testis possess astrocyte and oligodendrocyte marker molecules. Acta Histochemica, 104(1), 39–49. [ DOI ] [ PubMed ] [ Google Scholar ]
- Doerflinger N. H., Macklin W. B., Popko B. (2003). Inducible site-specific recombination in myelinating cells. Genesis (New York, N.Y.: 2000), 35(1), 63–72. [ DOI ] [ PubMed ] [ Google Scholar ]
- D’Souza W. N., Chang C. F., Fischer A. M., Li M., Hedrick S. M. (2008). The Erk2 MAPK regulates CD8 T cell proliferation and survival. Journal of Immunology (Baltimore, MD: 1950), 181(11), 7617–7629. [ DOI ] [ PMC free article ] [ PubMed ] [ Google Scholar ]
- Dumont F. J., Staruch M. J., Fischer P., DaSilva C., Camacho R. (1998). Inhibition of T cell activation by pharmacologic disruption of the MEK1/ERK MAP kinase or calcineurin signaling pathways results in differential modulation of cytokine production . Journal of Immunology (Baltimore, MD: 1950), 160(6), 2579–2589. [ PubMed ] [ Google Scholar ]
- Fillatreau S. (2019). Regulatory functions of B cells and regulatory plasma cells. Biomedical Journal, 42(4), 233–242. [ DOI ] [ PMC free article ] [ PubMed ] [ Google Scholar ]
- Funfschilling U., Supplie L. M., Mahad D., Boretius S., Saab A. S., Edgar J., Brinkmann B. G., Kassmann C. M., Tzvetanova I. D., Mobius W., Diaz F., Meijer D., Suter U., Hamprecht B., Sereda M. W., Moraes C. T., Frahm J., Goebbels S., Nave K. A. (2012). Glycolytic oligodendrocytes maintain myelin and long-term axonal integrity. Nature, 485(7399), 517–521. [ DOI ] [ PMC free article ] [ PubMed ] [ Google Scholar ]
- Fyffe-Maricich S. L., Schott A., Karl M., Krasno J., Miller R. H. (2013). Signaling through ERK1/2 controls myelin thickness during myelin repair in the adult central nervous system. The Journal of Neuroscience: The Official Journal of the Society for Neuroscience, 33(47), 18402–18408. [ DOI ] [ PMC free article ] [ PubMed ] [ Google Scholar ]
- Garaud S., Taher T. E., Debant M., Burgos M., Melayah S., Berthou C., Parikh K., Pers J. O., Luque-Paz D., Chiocchia G., Peppelenbosch M., Isenberg D. A., Youinou P., Mignen O., Renaudineau Y., Mageed R. A. (2018). CD5 expression promotes IL-10 production through activation of the MAPK/erk pathway and upregulation of TRPC1 channels in B lymphocytes. Cellular & Molecular Immunology, 15(2), 158–170. [ DOI ] [ PMC free article ] [ PubMed ] [ Google Scholar ]
- Gold M. R. (2008). B cell development: Important work for ERK. Immunity, 28(4), 488–490. [ DOI ] [ PubMed ] [ Google Scholar ]
- Guo J., Zhang J., Zhang X., Zhang Z., Wei X., Zhou X. (2014). Constitutive activation of MEK1 promotes treg cell instability in vivo. The Journal of Biological Chemistry, 289(51), 35139–35148. [ DOI ] [ PMC free article ] [ PubMed ] [ Google Scholar ]
- Hausser-Kinzel S., Weber M. S. (2019). The role of B cells and antibodies in multiple sclerosis, neuromyelitis optica, and related disorders. Frontiers in Immunology, 10, 201. [ DOI ] [ PMC free article ] [ PubMed ] [ Google Scholar ]
- Hussien Y., Cavener D. R., Popko B. (2014). Genetic inactivation of PERK signaling in mouse oligodendrocytes: Normal developmental myelination with increased susceptibility to inflammatory demyelination. Glia, 62(5), 680–691. [ DOI ] [ PMC free article ] [ PubMed ] [ Google Scholar ]
- Ishii A., Furusho M., Bansal R. (2013). Sustained activation of ERK1/2 MAPK in oligodendrocytes and schwann cells enhances myelin growth and stimulates oligodendrocyte progenitor expansion. The Journal of Neuroscience: The Official Journal of the Society for Neuroscience, 33(1), 175–186. [ DOI ] [ PMC free article ] [ PubMed ] [ Google Scholar ]
- Ishii A., Furusho M., Dupree J. L., Bansal R. (2016). Strength of ERK1/2 MAPK activation determines its effect on myelin and axonal integrity in the adult CNS. The Journal of Neuroscience: The Official Journal of the Society for Neuroscience, 36(24), 6471–6487. [ DOI ] [ PMC free article ] [ PubMed ] [ Google Scholar ]
- Ishii A., Fyffe-Maricich S. L., Furusho M., Miller R. H., Bansal R. (2012). ERK1/ERK2 MAPK signaling is required to increase myelin thickness independent of oligodendrocyte differentiation and initiation of myelination. The Journal of Neuroscience: The Official Journal of the Society for Neuroscience, 32(26), 8855–8864. [ DOI ] [ PMC free article ] [ PubMed ] [ Google Scholar ]
- Iyer S. S., Cheng G. (2012). Role of interleukin 10 transcriptional regulation in inflammation and autoimmune disease. Critical Reviews in Immunology, 32(1), 23–63. [ DOI ] [ PMC free article ] [ PubMed ] [ Google Scholar ]
- Jacob A., Cooney D., Pradhan M., Coggeshall K. M. (2002). Convergence of signaling pathways on the activation of ERK in B cells. The Journal of Biological Chemistry, 277(26), 23420–23426. [ DOI ] [ PubMed ] [ Google Scholar ]
- Jager A., Dardalhon V., Sobel R. A., Bettelli E., Kuchroo V. K. (2009). Th1, Th17, and Th9 effector cells induce experimental autoimmune encephalomyelitis with different pathological phenotypes. Journal of Immunology (Baltimore, MD: 1950), 183(11), 7169–7177. [ DOI ] [ PMC free article ] [ PubMed ] [ Google Scholar ]
- Jaini R., Popescu D. C., Flask C. A., Macklin W. B., Tuohy V. K. (2013). Myelin antigen load influences antigen presentation and severity of central nervous system autoimmunity . Journal of Neuroimmunology, 259(1-2), 37–46. [ DOI ] [ PMC free article ] [ PubMed ] [ Google Scholar ]
- Jeffries M. A., Urbanek K., Torres L., Wendell S. G., Rubio M. E., Fyffe-Maricich S. L. (2016). ERK1/2 activation in preexisting oligodendrocytes of adult mice drives new myelin synthesis and enhanced CNS function. The Journal of Neuroscience: The Official Journal of the Society for Neuroscience, 36(35), 9186–9200. [ DOI ] [ PMC free article ] [ PubMed ] [ Google Scholar ]
- Joseph S., Vingill S., Jahn O., Fledrich R., Werner H. B., Katona I., Mobius W., Mitkovski M., Huang Y., Weis J., Sereda M. W., Schulz J. B., Nave K. A., Stegmuller J. (2019). Myelinating glia-specific deletion of Fbxo7 in mice triggers axonal degeneration in the central nervous system together with peripheral neuropathy. The Journal of Neuroscience: The Official Journal of the Society for Neuroscience, 39(28), 5606–5626. [ DOI ] [ PMC free article ] [ PubMed ] [ Google Scholar ]
- Kang S. H., Fukaya M., Yang J. K., Rothstein J. D., Bergles D. E. (2010). NG2+ CNS glial progenitors remain committed to the oligodendrocyte lineage in postnatal life and following neurodegeneration. Neuron, 68(4), 668–681. [ DOI ] [ PMC free article ] [ PubMed ] [ Google Scholar ]
- Kubo M., Motomura Y. (2012). Transcriptional regulation of the anti-inflammatory cytokine IL-10 in acquired immune cells. Frontiers in Immunology, 3, 275. [ DOI ] [ PMC free article ] [ PubMed ] [ Google Scholar ]
- Lappe-Siefke C., Goebbels S., Gravel M., Nicksch E., Lee J., Braun P. E., Griffiths I. R., Nave K. A. (2003). Disruption of Cnp1 uncouples oligodendroglial functions in axonal support and myelination. Nature Genetics, 33(3), 366–374. [ DOI ] [ PubMed ] [ Google Scholar ]
- Lassmann H., Bradl M. (2017). Multiple sclerosis: Experimental models and reality. Acta Neuropathologica, 133(2), 223–244. [ DOI ] [ PMC free article ] [ PubMed ] [ Google Scholar ]
- Liu B. S., Cao Y., Huizinga T. W., Hafler D. A., Toes R. E. (2014). TLR-mediated STAT3 and ERK activation controls IL-10 secretion by human B cells. European Journal of Immunology, 44(7), 2121–2129. [ DOI ] [ PubMed ] [ Google Scholar ]
- Liu H., Yao S., Dann S. M., Qin H., Elson C. O., Cong Y. (2013). ERK differentially regulates Th17- and treg-cell development and contributes to the pathogenesis of colitis. European Journal of Immunology, 43(7), 1716–1726. [ DOI ] [ PubMed ] [ Google Scholar ]
- Luo X., Zhang Q., Liu V., Xia Z., Pothoven K. L., Lee C. (2008). Cutting edge: TGF-beta-induced expression of Foxp3 in T cells is mediated through inactivation of ERK . Journal of Immunology (Baltimore, MD: 1950), 180(5), 2757–2761. [ DOI ] [ PMC free article ] [ PubMed ] [ Google Scholar ]
- Lyons J. A., San M., Happ M. P., Cross A. H. (1999). B cells are critical to induction of experimental allergic encephalomyelitis by protein but not by a short encephalitogenic peptide. Eur J Immunol, 29(11), 3432–3439. [ DOI ] [ PubMed ] [ Google Scholar ]
- Madsen P. M., Motti D., Karmally S., Szymkowski D. E., Lambertsen K. L., Bethea J. R., Brambilla R. (2016). Oligodendroglial TNFR2 mediates membrane TNF-Dependent repair in experimental autoimmune encephalomyelitis by promoting oligodendrocyte differentiation and remyelination. The Journal of Neuroscience: The Official Journal of the Society for Neuroscience, 36(18), 5128–5143. [ DOI ] [ PMC free article ] [ PubMed ] [ Google Scholar ]
- Mann M. K., Ray A., Basu S., Karp C. L., Dittel B. N. (2012). Pathogenic and regulatory roles for B cells in experimental autoimmune encephalomyelitis. Autoimmunity, 45(5), 388–399. [ DOI ] [ PMC free article ] [ PubMed ] [ Google Scholar ]
- Matsumoto M., Baba A., Yokota T., Nishikawa H., Ohkawa Y., Kayama H., Kallies A., Nutt S. L., Sakaguchi S., Takeda K., Kurosaki T., Baba Y. (2014). Interleukin-10-producing plasmablasts exert regulatory function in autoimmune inflammation. Immunity, 41(6), 1040–1051. [ DOI ] [ PubMed ] [ Google Scholar ]
- Matsushita T., Horikawa M., Iwata Y., Tedder T. F. (2010). Regulatory B cells (B10 cells) and regulatory T cells have independent roles in controlling experimental autoimmune encephalomyelitis initiation and late-phase immunopathogenesis. Journal of Immunology (Baltimore, MD: 1950), 185(4), 2240–2252. [ DOI ] [ PMC free article ] [ PubMed ] [ Google Scholar ]
- Matsushita T., Yanaba K., Bouaziz J. D., Fujimoto M., Tedder T. F. (2008). Regulatory B cells inhibit EAE initiation in mice while other B cells promote disease progression. The Journal of Clinical Investigation, 118(10), 3420–3430. [ DOI ] [ PMC free article ] [ PubMed ] [ Google Scholar ]
- McFerran B., Burgoyne R. (1997). 2′,3′-Cyclic nucleotide 3′-phosphodiesterase is associated with mitochondria in diverse adrenal cell types. Journal of Cell Science, 110(Pt 23), 2979–2985. [ DOI ] [ PubMed ] [ Google Scholar ]
- Mei F., Lehmann-Horn K., Shen Y.-A A., Rankin K. A., Stebbins K. J., Lorrain D. S., Pekarek K., A Sagan S., Xiao L., Teuscher C., von Büdingen H.-C., Wess J., Lawrence J. J., Green A. J., Fancy S. P., Zamvil S. S., Chan J. R. (2016). Accelerated remyelination during inflammatory demyelination prevents axonal loss and improves functional recovery. Elife, 5 10.7554/eLife.18246 [ DOI ] [ PMC free article ] [ PubMed ] [ Google Scholar ]
- Mendel I., Kerlero de Rosbo N., Ben-Nun A. (1995). A myelin oligodendrocyte glycoprotein peptide induces typical chronic experimental autoimmune encephalomyelitis in H-2b mice: Fine specificity and T cell receptor V beta expression of encephalitogenic T cells. European Journal of Immunology, 25(7), 1951–1959. [ DOI ] [ PubMed ] [ Google Scholar ]
- Meyer N., Richter N., Fan Z., Siemonsmeier G., Pivneva T., Jordan P., Steinhauser C., Semtner M., Nolte C., Kettenmann H. (2018). Oligodendrocytes in the mouse corpus callosum maintain axonal function by delivery of glucose. Cell Reports, 22(9), 2383–2394. [ DOI ] [ PubMed ] [ Google Scholar ]
- Michel K., Zhao T., Karl M., Lewis K., Fyffe-Maricich S. L. (2015). Translational control of myelin basic protein expression by ERK2 MAP kinase regulates timely remyelination in the adult brain. The Journal of Neuroscience: The Official Journal of the Society for Neuroscience, 35(20), 7850–7865. [ DOI ] [ PMC free article ] [ PubMed ] [ Google Scholar ]
- Miyoshi K., Cui Y., Riedlinger G., Robinson P., Lehoczky J., Zon L., Oka T., Dewar K., Hennighausen L. (2001). Structure of the mouse stat 3/5 locus: Evolution from drosophila to zebrafish to mouse. Genomics, 71(2), 150–155. [ DOI ] [ PubMed ] [ Google Scholar ]
- Muzumdar M. D., Tasic B., Miyamichi K., Li L., Luo L. (2007). A global double-fluorescent cre reporter mouse. Genesis (New York, N.Y.: 2000), 45(9), 593–605. [ DOI ] [ PubMed ] [ Google Scholar ]
- Nishizawa Y., Kurihara T., Masuda T., Takahashi Y. (1985). Immunohistochemical localization of 2′,3′-cyclic nucleotide 3′-phosphodiesterase in adult bovine cerebrum and cerebellum. Neurochemical Research, 10(8), 1107–1118. [ DOI ] [ PubMed ] [ Google Scholar ]
- Okuda Y., Sakoda S., Fujimura H., Saeki Y., Kishimoto T., Yanagihara T. (1999). IL-6 plays a crucial role in the induction phase of myelin oligodendrocyte glucoprotein 35-55 induced experimental autoimmune encephalomyelitis. Journal of Neuroimmunology, 101(2), 188–196. 10.1016/S0165-5728(99)00139-3 [ DOI ] [ PubMed ] [ Google Scholar ]
- Pachynski R. K., Scholz A., Monnier J., Butcher E. C., Zabel B. A. (2015). Evaluation of tumor-infiltrating leukocyte subsets in a subcutaneous tumor model. Journal of Visualized Experiments, 98, e52657. [ DOI ] [ PMC free article ] [ PubMed ] [ Google Scholar ]
- Parker Harp C. R., Archambault A. S., Sim J., Ferris S. T., Mikesell R. J., Koni P. A., Shimoda M., Linington C., Russell J. H., Wu G. F. (2015). B cell antigen presentation is sufficient to drive neuroinflammation in an animal model of multiple sclerosis. Journal of Immunology (Baltimore, MD: 1950), 194(11), 5077–5084. [ DOI ] [ PMC free article ] [ PubMed ] [ Google Scholar ]
- Pennati A., Ng S., Wu Y., Murphy J. R., Deng J., Rangaraju S., Asress S., Blanchfield J. L., Evavold B., Galipeau J. (2016). Regulatory B cells induce formation of IL-10-Expressing T cells in mice with autoimmune neuroinflammation. The Journal of Neuroscience: The Official Journal of the Society for Neuroscience, 36(50), 12598–12610. [ DOI ] [ PMC free article ] [ PubMed ] [ Google Scholar ]
- Pennati A., Nylen E. A., Duncan I. D., Galipeau J. (2020). Regulatory B cells normalize CNS myeloid cell content in a mouse model of multiple sclerosis and promote oligodendrogenesis and remyelination. The Journal of Neuroscience: The Official Journal of the Society for Neuroscience, 40(26), 5105–5115. [ DOI ] [ PMC free article ] [ PubMed ] [ Google Scholar ]
- Pierson E. R., Stromnes I. M., Goverman J. M. (2014). B cells promote induction of experimental autoimmune encephalomyelitis by facilitating reactivation of T cells in the central nervous system. Journal of Immunology (Baltimore, MD: 1950), 192(3), 929–939. [ DOI ] [ PMC free article ] [ PubMed ] [ Google Scholar ]
- Pollok K., Mothes R., Ulbricht C., Liebheit A., Gerken J. D., Uhlmann S., Paul F., Niesner R., Radbruch H., Hauser A. E. (2017). The chronically inflamed central nervous system provides niches for long-lived plasma cells. Acta Neuropathologica Communications, 5(1), 88. [ DOI ] [ PMC free article ] [ PubMed ] [ Google Scholar ]
- Rangachari M., Kuchroo V. K. (2013). Using EAE to better understand principles of immune function and autoimmune pathology. Journal of Autoimmunity, 45, 31–39. [ DOI ] [ PMC free article ] [ PubMed ] [ Google Scholar ]
- Rao K. M. (2001). MAP kinase activation in macrophages. Journal of Leukocyte Biology, 69(1), 3–10. [ PubMed ] [ Google Scholar ]
- Ray A., Basu S. (2014). Regulatory B cells in experimental autoimmune encephalomyelitis (EAE). Methods in Molecular Biology (Clifton, N.J.), 1190, 243–255. [ DOI ] [ PubMed ] [ Google Scholar ]
- Rayasam A., Kijak J. A., Dallmann M., Hsu M., Zindl N., Lindstedt A., Steinmetz L., Harding J. S., Harris M. G., Karman J., Sandor M., Fabry Z. (2018). Regional distribution of CNS antigens differentially determines T-Cell mediated neuroinflammation in a CX3CR1-Dependent manner. The Journal of Neuroscience: The Official Journal of the Society for Neuroscience, 38(32), 7058–7071. [ DOI ] [ PMC free article ] [ PubMed ] [ Google Scholar ]
- Ren Z., Wang Y., Tao D., Liebenson D., Liggett T., Goswami R., Clarke R., Stefoski D., Balabanov R. (2011). Overexpression of the dominant-negative form of interferon regulatory factor 1 in oligodendrocytes protects against experimental autoimmune encephalomyelitis. The Journal of Neuroscience: The Official Journal of the Society for Neuroscience, 31(23), 8329–8341. [ DOI ] [ PMC free article ] [ PubMed ] [ Google Scholar ]
- Richards J. D., Dave S. H., Chou C. H., Mamchak A. A., DeFranco A. L. (2001). Inhibition of the MEK/ERK signaling pathway blocks a subset of B cell responses to antigen . Journal of Immunology (Baltimore, MD: 1950), 166(6), 3855–3864. [ DOI ] [ PubMed ] [ Google Scholar ]
- Rojas O. L., Pröbstel A.-K., Porfilio E. A., Wang A. A., Charabati M., Sun T., Lee D. S. W., Galicia G., Ramaglia V., Ward L. A., Leung L. Y. T., Najafi G., Khaleghi K., Garcillán B., Li A., Besla R., Naouar I., Cao E. Y., Chiaranunt P., . . . Gommerman J. L. (2019). Recirculating intestinal IgA-Producing cells regulate neuroinflammation via IL-10. Cell, 177(2), 492–493. [ DOI ] [ PubMed ] [ Google Scholar ]
- Rui L., Healy J. I., Blasioli J., Goodnow C. C. (2006). ERK signaling is a molecular switch integrating opposing inputs from B cell receptor and T cell cytokines to control TLR4-driven plasma cell differentiation . Journal of Immunology (Baltimore, MD: 1950), 177(8), 5337–5346. [ DOI ] [ PubMed ] [ Google Scholar ]
- Sabeur G., Wantyghem J., Schuller E. (1986). Stimulation of 2′,3′-cyclic nucleotide 3′-phosphodiesterase in human lymphocytes by robinia pseudoacacia lectin. Biochimie, 68(4), 581–585. [ DOI ] [ PubMed ] [ Google Scholar ]
- Samoilova E. B., Horton J. L., Hilliard B., Liu T. S., Chen Y. (1998). IL-6-deficient mice are resistant to experimental autoimmune encephalomyelitis: Roles of IL-6 in the activation and differentiation of autoreactive T cells. Journal of Immunology (Baltimore, MD: 1950), 161(12), 6480–6486. [ PubMed ] [ Google Scholar ]
- Sanin D. E., Prendergast C. T., Mountford A. P. (2015). IL-10 production in macrophages is regulated by a TLR-Driven CREB-Mediated mechanism that is linked to genes involved in cell metabolism. Journal of Immunology (Baltimore, MD: 1950), 195(3), 1218–1232. [ DOI ] [ PMC free article ] [ PubMed ] [ Google Scholar ]
- Saraiva M., O’Garra A. (2010). The regulation of IL-10 production by immune cells. Nature Reviews. Immunology, 10(3), 170–181. [ DOI ] [ PubMed ] [ Google Scholar ]
- Serada S., Fujimoto M., Mihara M., Koike N., Ohsugi Y., Nomura S., Yoshida H., Nishikawa T., Terabe F., Ohkawara T., Takahashi T., Ripley B., Kimura A., Kishimoto T., Naka T. (2008). IL-6 blockade inhibits the induction of myelin antigen-specific Th17 cells and Th1 cells in experimental autoimmune encephalomyelitis. Proceedings of the National Academy of Sciences of the United States of America, 105(26), 9041–9046. [ DOI ] [ PMC free article ] [ PubMed ] [ Google Scholar ]
- Sheedlo H. J., Doran J. E., Sprinkle T. J. (1984). An investigation of 2′:3′-cyclic nucleotide 3′-phosphodiesterase (EC 3.1.4.37, CNP) in peripheral blood elements and CNS myelin. Life Sciences, 34(18), 1731–1737. 10.1016/0024-3205(84)90572-1 [ DOI ] [ PubMed ] [ Google Scholar ]
- Shen P., Roch T., Lampropoulou V., O'Connor R. A., Stervbo U., Hilgenberg E., Ries S., Dang V. D., Jaimes Y., Daridon C., Li R., Jouneau L., Boudinot P., Wilantri S., Sakwa I., Miyazaki Y., Leech M. D., McPherson R. C., Wirtz S., . . . Fillatreau S. (2014). IL-35-producing B cells are critical regulators of immunity during autoimmune and infectious diseases. Nature, 507(7492), 366–370. [ DOI ] [ PMC free article ] [ PubMed ] [ Google Scholar ]
- Simons M., Nave K. A. (2015). Oligodendrocytes: Myelination and axonal support. Cold Spring Harbor Perspectives in Biology, 8(1), a020479. [ DOI ] [ PMC free article ] [ PubMed ] [ Google Scholar ]
- Srinivasan L., Sasaki Y., Calado D. P., Zhang B., Paik J. H., DePinho R. A., Kutok J. L., Kearney J. F., Otipoby K. L., Rajewsky K. (2009). PI3 kinase signals BCR-dependent mature B cell survival. Cell, 139(3), 573–586. [ DOI ] [ PMC free article ] [ PubMed ] [ Google Scholar ]
- Sutavani R. V., Phair I. R., Barker R., McFarlane A., Shpiro N., Lang S., Woodland A., Arthur J. S. C. (2018). Differential control of toll-like receptor 4-induced interleukin-10 induction in macrophages and B cells reveals a role for p90 ribosomal S6 kinases. The Journal of Biological Chemistry, 293(7), 2302–2317. [ DOI ] [ PMC free article ] [ PubMed ] [ Google Scholar ]
- Teodorovic L. S., Babolin C., Rowland S. L., Greaves S. A., Baldwin D. P., Torres R. M., Pelanda R. (2014). Activation of ras overcomes B-cell tolerance to promote differentiation of autoreactive B cells and production of autoantibodies. Proceedings of the National Academy of Sciences of the United States of America, 111(27), E2797–2806. [ DOI ] [ PMC free article ] [ PubMed ] [ Google Scholar ]
- Terry R. L., Ifergan I., Miller S. D. (2016). Experimental autoimmune encephalomyelitis in mice. Methods in Molecular Biology (Clifton, N.J.), 1304, 145–160. [ DOI ] [ PMC free article ] [ PubMed ] [ Google Scholar ]
- Trapp B. D., Bernier L., Andrews S. B., Colman D. R. (1988). Cellular and subcellular distribution of 2′,3′-cyclic nucleotide 3′-phosphodiesterase and its mRNA in the rat Central nervous system . Journal of Neurochemistry, 51(3), 859–868. [ DOI ] [ PubMed ] [ Google Scholar ]
- Traves P. G., de Atauri P., Marin S., Pimentel-Santillana M., Rodriguez-Prados J. C., Marin de Mas I., Selivanov V. A., Martin-Sanz P., Bosca L., Cascante M. (2012). Relevance of the MEK/ERK signaling pathway in the metabolism of activated macrophages: A metabolomic approach. Journal of Immunology (Baltimore, MD: 1950), 188(3), 1402–1410. [ DOI ] [ PubMed ] [ Google Scholar ]
- Weissbarth S., Maker H. S., Raes I., Brannan T. S., Lapin E. P., Lehrer G. M. (1981). The activity of 2′,3′-cyclic nucleotide 3′-phosphodiesterase in rat tissues. Journal of Neurochemistry, 37(3), 677–680. [ DOI ] [ PubMed ] [ Google Scholar ]
- Wujek J. R., Bjartmar C., Richer E., Ransohoff R. M., Yu M., Tuohy V. K., Trapp B. D. (2002). Axon loss in the spinal cord determines permanent neurological disability in an animal model of multiple sclerosis. Journal of Neuropathology and Experimental Neurology, 61(1), 23–32. [ DOI ] [ PubMed ] [ Google Scholar ]
- Xiao B. G., Bai X. F., Zhang G. X., Link H. (1998). Suppression of acute and protracted-relapsing experimental allergic encephalomyelitis by nasal administration of low-dose IL-10 in rats. Journal of Neuroimmunology, 84(2), 230–237. [ DOI ] [ PubMed ] [ Google Scholar ]
- Yanaba K., Bouaziz J. D., Haas K. M., Poe J. C., Fujimoto M., Tedder T. F. (2008). A regulatory B cell subset with a unique CD1dhiCD5+ phenotype controls T cell-dependent inflammatory responses. Immunity, 28(5), 639–650. [ DOI ] [ PubMed ] [ Google Scholar ]
- Yue F., Cheng Y., Breschi A., Vierstra J., Wu W., Ryba T., Sandstrom R., Ma Z., Davis C., Pope B. D., Shen Y., Pervouchine D. D., Djebali S., Thurman R. E., Kaul R., Rynes E., Kirilusha A., Marinov G. K., Williams B. A., … Ren B., Mouse ENCODE Consortium (2014). A comparative encyclopedia of DNA elements in the mouse genome. Nature, 515(7527), 355–364. [ DOI ] [ PMC free article ] [ PubMed ] [ Google Scholar ]
- Yue Y., Stanojlovic M., Lin Y., Karsenty G., Lin W. (2019). Oligodendrocyte-specific ATF4 inactivation does not influence the development of EAE . Journal of Neuroinflammation, 16(1), 23. [ DOI ] [ PMC free article ] [ PubMed ] [ Google Scholar ]
- Zhang H., Kuchroo V. (2019). Epigenetic and transcriptional mechanisms for the regulation of IL-10. Seminars in Immunology, 44, 101324. [ DOI ] [ PMC free article ] [ PubMed ] [ Google Scholar ]
- View on publisher site
- PDF (1.7 MB)
- Collections
Similar articles
Cited by other articles, links to ncbi databases.
- Download .nbib .nbib
- Format: AMA APA MLA NLM
Add to Collections
- Open access
- Published: 26 May 2021
miR-20a suppresses Treg differentiation by targeting Map3k9 in experimental autoimmune encephalomyelitis
- Yishu Wang ORCID: orcid.org/0000-0002-3897-6370 1 ,
- Chong Xie 1 ,
- Yaying Song 1 ,
- Weiwei Xiang 1 ,
- Jing Peng 1 ,
- Jie Ding 1 &
- Yangtai Guan 1
Journal of Translational Medicine volume 19 , Article number: 223 ( 2021 ) Cite this article
2237 Accesses
5 Citations
1 Altmetric
Metrics details
Experimental autoimmune encephalomyelitis (EAE) is a model for inflammatory demyelinating diseases of the central nervous system (CNS), a group of autoimmune diseases characterized by inflammatory infiltration, demyelination, and axonal damage. miR-20a is dysregulated in patients with CNS inflammatory demyelinating diseases; however, the function of miR-20a remains unclear. In this study, we intended to explore the role of miR-20a in EAE.
The expression of miR-20a was detected by quantitative real-time PCR (qRT-PCR) in EAE mice and patients with MOG antibody-associated demyelinating diseases. CD4 + T cells of EAE mice were sorted, stimulated, and polarized with miR-20a knockdown. Activation and differentiation of CD4 + T cells were analyzed by flow cytometry . The expression of target gene Map3k9 was detected by qRT-PCR and western blot experiments. The binding of miR-20a to the 3’ UTR of Map3k9 was tested by luciferase assays . The feasibility of miR-20a as a therapeutic target to alleviate the severity of EAE was explored by intravenous administration of miR-20a antagomirs to EAE mice.
miR-20a was upregulated in splenocytes and lymph node cells, CD4 + T cells, and spinal cords of EAE mice. Moreover, miR-20a knockdown did not influence the activation of antigen-specific CD4 + T cells but promoted their differentiation into Treg cells. Map3k9 was predicted to be a target gene of miR-20a. The expressions of Map3k9 and miR-20a were negatively correlated, and miR-20a knockdown increased the expression of Map3k9. In addition, miR-20a binded to the 3’ UTR of Map3k9 , and simultaneous knockdown of miR-20a and Map3k9 counteracted the enhanced differentiation of Tregs observed when miR-20a was knocked down alone. Furthermore, injection of miR-20a antagomirs to EAE mice reduced the severity of the disease and increased the proportion of Treg cells in peripheral immune organs.
Conclusions
miR-20a suppresses the differentiation of antigen-specific CD4 + T cells into Tregs in EAE by decreasing the expression of Map3k9. miR-20a antagomirs alleviate EAE, suggesting a new therapy for EAE and CNS inflammatory demyelinating diseases.
Inflammatory demyelinating diseases of the central nervous system (CNS) are a group of autoimmune diseases targeting oligodendrocytes or support cells (such as astrocytes); these diseases are characterized by inflammatory infiltration, demyelination, and axonal damage, and examples include multiple sclerosis (MS), neuromyelitis optica spectrum disorder (NMOSD), and myelin oligodendrocyte glycoprotein (MOG) antibody-associated demyelinating disease [ 1 , 2 , 3 , 4 ]. Millions of patients worldwide suffer various symptoms, including visual, motor, and sensory disturbances [ 5 ], while therapies for these diseases are still quite limited.
To date, the exact mechanism resulting in CNS inflammatory demyelinating diseases is still unclear, and both innate and adaptive immune cells have been reported to be involved [ 6 , 7 , 8 ]. Most studies on the pathogenesis of CNS inflammatory demyelinating diseases have been carried out on an experimental autoimmune encephalomyelitis (EAE) model. EAE used to be the most widely accepted model for MS [ 9 , 10 ]; however, in recent years, a growing number of studies have demonstrated that the disease which EAE reproduces is MOG antibody-associated demyelinating disease [ 11 , 12 ]. Although the mechanism for the development of EAE is uncertain, autoreactive CD4 + T cells are generally recognized as the main cells mediating myelin damage [ 13 , 14 ]. In the EAE model, IFN-γ-producing T helper (Th)1 and IL-17A-producing Th17 cells are considered crucial effector cells [ 9 , 10 , 15 ]. They infiltrate into CNS lesions in both EAE mice and patients [ 9 , 10 ], and transplanted autoreactive Th1 and Th17 cells can induce EAE symptoms in wild-type (WT) recipient mice. They activate astrocytes and microglia and secrete proinflammatory cytokines such as IFN-γ, IL-17A/F, and GM-CSF to cause tissue damage [ 5 , 9 , 10 , 13 , 14 , 16 , 17 , 18 ]. In contrast, adoptive transfer of CD25 + Foxp3 + regulatory T (Treg) cells is able to ameliorate EAE symptoms, whereas the depletion of Tregs worsens the disease [ 19 ], indicating the essential role of Tregs in the suppression of the autoimmune response and maintenance of immune tolerance.
MicroRNAs (miRNAs) are a class of non-coding RNA molecules that play a necessary role in cell differentiation, proliferation, development, and survival by binding to the complementary 3’ UTR of target mRNAs, resulting in mRNA translational inhibition or degradation [ 20 , 21 ]. In addition, miRNAs are frequently transcribed together as polycistronic primary transcripts that are processed into multiple individual mature miRNAs that are coordinated in function [ 22 ]. The miR-17–92 cluster is a typical polycistronic miRNA gene encoding 6 miRNAs (miR-17, miR-18a, miR-19a, miR-20a, miR-19b-1, and miR-92a-1). Identified in 2005, miR-17–92 was initially distinguished as an ‘oncomir’ because of its oncogenic nature in hematopoietic malignancies, medulloblastomas, lung cancer, colon cancer, and pancreatic cancer [ 23 , 24 ]. However, in recent years, the effects of miR-17–92 on CD4 + T cells have been gradually revealed. Overexpressing miR-17–92 in CD4 + T cells results in a higher percentage of cells in the S phase when stimulated with antigen [ 25 ] and a lower percentage of cells in the Sub-G0 phase when stimulated with anti-CD3 [ 26 ], indicating that miR-17–92 promotes proliferation and survival of activated CD4 + T cells. In addition, naïve CD4 + T cells deficient in miR-17–92 differentiate into fewer Th1 and Th17 cells but more induced Treg (iTreg) cells, suggesting the important role of miR-17–92 in the differentiation of CD4 + T cells [ 27 , 28 ]. Furthermore, individual miRNAs of miR-17–92 are upregulated or downregulated in the peripheral blood of patients, and the expressions of the miRNAs revert to normal levels with medical treatment or during remission [ 29 , 30 , 31 , 32 , 33 , 34 ]. In general, accumulating evidence has demonstrated that miR-17–92 is an essential regulator of CD4 + T cells and that the expression of miR-17–92 is altered in patients with CNS inflammatory demyelinating diseases; however, the precise role of miR-17–92 in the development of diseases and whether miR-17–92 affects diseases by modulating CD4 + T cells remain unknown.
In this study, we focused on miR-20a, a member of the miR-17–92 cluster. We first detected the upregulation of miR-20a in EAE mice and patients with MOG antibody-associated demyelinating diseases. We then explored the role of miR-20a in the activation and differentiation of antigen-specific CD4 + T cells in EAE. In addition, we found that Map3k9 (mitogen-activated protein kinase kinase kinase 9) is the target gene of miR-20a in CD4 + T cells of EAE mice, as confirmed by luciferase assays and functional validation. Furthermore, we tested the therapeutic potential of miR-20a antagomirs and found that miR-20a antagomirs alleviated EAE.
C57BL/6 mice were purchased from Lingchang Biotechnology Company (Shanghai, China). The animals were housed and fed in a specific pathogen-free animal facility at the Experimental Animal Center of Renji Hospital. 8 to 12-week-old female mice were used for all experiments. Experiments were performed in accordance with the guidelines for animal care and were approved by the Animal Ethics and Welfare Committee of Renji Hospital affiliated to Shanghai Jiaotong University School of Medicine (Shanghai, China).
EAE induction and evaluation
For EAE induction, myelin oligodendrocyte glycoprotein residues 35–55 (MOG 35–55 ) peptide (Met-Glu-Val-Gly-Trp-Tyr-Arg-Ser-Pro-Phe-Ser-Arg-Val-Val-His-Leu-Tyr-Arg-Asn-Gly-Lys, GL Biochem Ltd, Shanghai, China) dissolved in PBS was emulsified with complete Freund’s adjuvant (CFA), which is composed of incomplete Freund’s adjuvant and Mycobacterium tuberculosis H37Ra (BD Difco, MI, USA). The final concentrations of MOG 35-55 peptide and Mycobacterium tuberculosis H37Ra in the MOG 35-55 /CFA emulsion were 1.5 mg/ml and 4 mg/ml, respectively. Each mouse was injected subcutaneously with 200 μl MOG 35–55 /CFA emulsion near bilateral inguinal lymph nodes. In addition, 200 ng pertussis toxin (PTX; Millipore, Billerica, MA, USA) was administered intraperitoneally on the day of immunization (Day 0) and again 2 days later (Day 2).
Clinical assessment of EAE was performed daily after disease induction according to the following scoring system: 0, no clinical symptoms; 0.5, limp tail; 1, paralyzed tail; 1.5, hindlimb weakness, uncoordinated movement; 2, hindlimb paresis; 2.5, paralysis of one hindlimb; 3, paralysis of one hindlimb with the other hindlimb weakness; 3.5, complete paralysis of both hindlimbs; 4, hindlimb paralysis with forelimb paresis; 4.5, forelimb and hindlimb paralysis; and 5, moribund state or death.
RNA extraction, reverse transcription, and quantitative real-time PCR
Total RNA (including mRNA and small RNA) was extracted from cell pellets and spinal cord tissues using an RNeasy Mini Kit (QIAGEN, Hilden, Germany) according to the manufacturer’s instructions. Reverse transcription was performed using a Mir-X™ miRNA First Strand Synthesis Kit (Cat. 638315, Takara, Shiga, Japan) for miRNA and a PrimeScript™ RT reagent Kit (Cat. RR037A, Takara, Shiga, Japan) for mRNA. For relative quantitative real-time PCR (qRT-PCR), SYBR Advantage qPCR Premix (Cat. 639676, Takara, Shiga, Japan) and SYBR Green master mix (Cat. RR820A, Takara, Shiga, Japan) were used for the cDNA of miRNA and mRNA following the manufacturer’s instructions. The reactions were performed in a LightCycler 480 System (Roche, Basel, Switzerland), and U6 snRNA and β-actin were used as endogenous controls of miRNA and mRNA, respectively. The expression of the miRNAs and mRNAs normalized to the endogenous control were calculated using the 2 −ΔΔCT method and are presented as the fold change relative to the control group. The primer sequences applied in this study are listed in Additional file 1 : Table S1.
Isolation of CD4 + T cells
For isolation of CD4 + T cells from EAE and control mice, mice were sacrificed at the peak stage of disease after immunization, and the spleens and lymph nodes were harvested. The tissues were ground on a 40-μm strainer to prepare single-cell suspensions. After lysis of erythrocytes, splenocytes and lymph node cells were pelleted to isolate CD4 + T cells by magnetic bead cell sorting (MACS) according to the manufacturer’s instructions (Miltenyi Biotech, Bergisch Gladbach, Germany). In brief, the cell pellet was resuspended in MACS buffer and incubated with biotin-antibody cocktail for 5 min at 2–8 °C. After that, anti-biotin microbeads were added, for isolation of naïve CD4 + T cells, CD44 microbeads were also added. Then the mixture was incubated for 10 min at 2–8 °C. After incubation, the cells were separated with an LS Column in a MACS Separator, and the unlabeled cells in the flow-through, which are the CD4 + T cells, were collected.
Transfection of antagomirs and small interfering RNAs
For miR-20a and Map3k9 silencing, a chemically modified ssRNA oligonucleotide, miR-20a antagomir, was applied to knock down the expression of miR-20a, and small interfering RNA (siRNA) duplexes of Map3k9 were used for the knockdown of Map3k9. The antagomirs, siRNAs, and negative control were synthesized by GenePharma Ltd. (Shanghai, China), and their sequences were as follows. miR-20a antagomir: 5’-CUACCUGCACUAUAAGCACUUUA-3’; Map3k9 siRNA: sense, 5’-GGACCAGCUAACGACUAUATT -3’, antisense, 5’- UAUAGUCGUUAGCUGGUCCTT -3’; NC antagomir: 5’- CAGUACUUUUGUGUAGUACAA -3’. The CD4 + T cells isolated from splenocytes and lymph node cells were transfected with the miR-20a antagomirs (200 nM), Map3k9 siRNA (400 nM), or negative control using Entranster™-R4000 (Cat. 4000-3, Engreen, Beijing, China) and harvested 12 h after transfection for the following experiments.
Treg polarization of naïve or MOG-specific CD4 + T cells in vitro
Cells were cultured in complete RPMI medium (10% fetal bovine serum, 2 mM GlutaMAX™ Supplement (Thermo Fisher Scientific, Waltham, MA, USA), 25 mM HEPES, 55 μM 2-mercaptoethanol, mycoplasma prophylactic reagent, and penicillin/streptomycin) in 24-well plates (3 × 10 6 cells/ml). To induce the differentiation of naïve CD4 + T cells into Treg cells, an anti-CD3e (145-2C11, 10 μg/ml, Thermo Fisher Scientific, Waltham, MA, USA) antibody and anti-CD28 antibody (37.51, 10 μg/ml, Thermo Fisher Scientific, Waltham, MA, USA) were used to stimulate the cells, and recombinant mouse IL-2 (20 ng/ml, R&D Systems, Minneapolis, MN, USA) and mouse TGF-β1 (20 ng/ml, PeproTech, Rocky Hill, NJ, USA) were added to the medium for Treg polarization. Naïve CD4 + T cells stimulated with anti-CD3 and anti-CD28 antibodies without IL-2 or TGF-β1 were cultured as a control. The cells were polarized for 4 days before harvest.
To polarize MOG-specific CD4 + T cells into Treg cells, CD4 + T cells isolated from EAE were mixed with the remaining splenocytes and lymph node cells after transfection. MOG 35–55 (40 μg/ml, GL Biochem Ltd, Shanghai, China) was used to stimulate the cells in the culture medium, and recombinant mouse IL-2 (20 ng/ml, R&D Systems, Minneapolis, MN, USA) and mouse TGF-β1 (20 ng/ml, PeproTech, Rocky Hill, NJ, USA) were added for Treg polarization. The cells were polarized for 5 days before harvesting.
Flow cytometry
To analyze the activation and polarization of CD4 + T cells, the cells were first cultured for 3 to 5 days with stimulation as mentioned above. In some situations, the cells were restimulated with a cell stimulation cocktail (plus protein transport inhibitors) (Thermo Fisher Scientific, Waltham, MA, USA) for 5 h before harvesting if intracellular staining for cytokines was needed. For flow staining, the cells were first surface stained with antibodies against CD4, CD69, CD62L, and CD25 conjugated to fluorochromes, and then the cells were fixed and permeabilized with the Foxp3/Transcription Factor Staining Buffer Set (Thermo Fisher Scientific, Waltham, MA, USA). Then, intracellular staining for Foxp3, IFN-γ, and IL-17A was performed, followed by fixation using 2% paraformaldehyde. Isotype plus fluorescence minus one (FMO) controls were used for gating and nonspecific staining exclusion. All antibodies used in this study were purchased from BD Biosciences. Data were acquired on a BD LSRFortessa X-20 or BD Accuri C6 Plus instrument and analyzed with FlowJo software.
Western blot
Spinal cord tissues were lysed with RIPA lysis buffer (Beyotime, Shanghai, China) containing protease and phosphatase inhibitors (Beyotime, Shanghai, China) and 5 mM EDTA to extract total protein. Protein concentration was measured using a Pierce™ BCA Protein Assay Kit (Thermo Fisher Scientific, Waltham, MA, USA). Equal amounts of protein (20 μg) for each sample were separated by 8% SDS-PAGE and transferred onto polyvinylidene fluoride (PVDF) membranes. Nonspecific binding was blocked by 5% BSA for 2 h at room temperature. The membranes were then incubated with anti-MLK1 (Map3k9) antibody (1:1000, Cat. #5029, CST, Danvers, MA, USA) and anti-β-actin antibody (1:2000, Cat. WB0196, Weiao, Shanghai, China) overnight at 4 °C, followed by incubation with HRP-conjugated secondary antibodies for 2 h at room temperature. Blots were visualized using ECL substrate (Weiao, Shanghai, China) on a ChemiDoc™ imaging system (Bio‐Rad, Danvers, MA, USA).
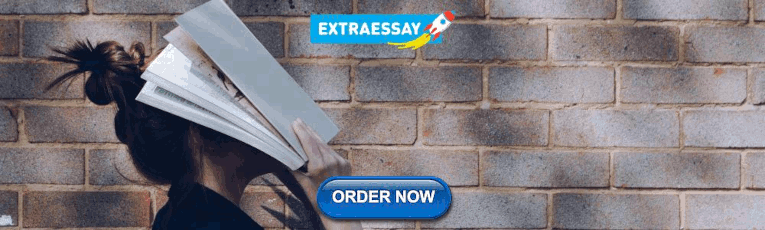
miR-20a knockdown using antagomirs in vivo
To knock down the expression of miR-20a, chemically modified single-stranded miR-20a antagomirs (purchased from GenePharma Ltd, Shanghai, China) were used. miR-20a antagomirs in the transfection complexes were delivered by tail vein injection to EAE mice. The transfection complexes were prepared with Entranster™-in vivo (Engreen, Beijing, China) according to the manufacturer’s instructions. The miR-20a antagomirs were administered intravenously for 3 days from the day that clinical symptoms appeared (onset stage). For each mouse, 100 μg miR-20a antagomirs were injected for the first two days, and 50 μg was injected for the third day.
3’ UTR luciferase assay
The wild-type 3’ UTR of Map3k9 containing the predicted binding site, mutant 3' UTR, or NC 3' UTR was amplified by PCR and cloned into a luciferase reporter vector (PGL3-CMV-LUC-MCS; Genomeditech, Shanghai, China) by Genomeditech Co., Ltd. Each vector, along with a Renilla luciferase vector (pRL-TK) and miR-20a mimics or control NC mimics, was transfected into HEK-293 cells using HG transgene reagent (Genomeditech, Shanghai, China). Cells were harvested and lysed at 48 h post-transfection. The luciferase activity was measured by an Infinite M1000 plate reader (Tecan, Switzerland), and the results are shown as relative light units (RLUs). The RLU of luciferase reporter containing 3’ UTR/RLU of Renilla luciferase ratios were normalized to that of cells cotransfected with luciferase reporter vector containing NC 3’ UTR, NC mimics, and pRL-TK. The values of the normalized RLU ratios were used for statistical analysis.
Histological analysis
The spinal cords harvested from EAE mice were first fixed with 4% paraformaldehyde, and then the lumbar enlargements were embedded in paraffin and cut into 5-μm-thick sections, followed by hematoxylin and eosin (H&E) and Luxol fast blue staining. The number of infiltrating inflammatory cells was counted in the H&E-stained sections to represent the severity of inflammation, while the demyelination severity is expressed as the percentage of demyelinated area to total white matter area in Luxol fast blue stained sections.
Statistical analysis
Statistical analysis was carried out using GraphPad Prism software (Version 8.3.1). The Shapiro–Wilk test was used for normality analysis of the data distribution. The unpaired Student’s t -test or paired Student’s t -test was used for significant difference analysis between two groups. The correlation between the expression of miR-20a and Map3k9 was identified with simple linear regression analysis. To compare the severity of disease in the two groups of EAE mice over the entire period of the disease, the area under the curve (RUC) for each mouse was calculated, and the difference was identified with Student’s t -test. In addition, to compare the severity of disease on each individual day, the clinical scores of the EAE mice from the two groups were analyzed by the Mann–Whitney test. Data are expressed as the mean ± SEM, and a p -value < 0.05 was considered significant.
miR-20a is increased in EAE mice and patients with MOG antibody-associated demyelinating diseases
To determine whether the miR-17-92 cluster is involved in the inflammatory response during EAE progression, we first tested the expression of the 6 miRNAs in the miR-17-92 cluster in splenocytes and lymph node cells. Compared to the control group, the expression levels of miR-17, miR-18a, miR-20a, and miR-92a-1 were increased in EAE mice, while miR-19a and miR-19b-1 were expressed equivalently between the two groups (Fig. 1 A). We next detected the expression of miR-17, miR-18a, miR-20a, and miR-92a-1 in CD4 + T cells isolated from EAE and control mice, and miR-17 and miR-20a were found to be upregulated in EAE mice (Fig. 1 B). Furthermore, the same results for miR-17 and miR-20a were also observed in spinal cord tissue of EAE and control mice (Fig. 1 C). To determine the importance of the miRNAs in patients, we tested the expression level of the miRNAs in peripheral blood leukocytes from patients with MOG antibody-associated demyelinating diseases. Increased expression levels of miR-17, miR-18a, miR-20a, and miR-92a-1 were detected in peripheral blood leukocytes from patients compared with healthy controls (Fig. 1 D), which were consistent with the results in splenocytes and lymph node cells from EAE mice. The above findings indicated that miR-17-92 cluster may be involved in inflammatory demyelinating diseases of the CNS. Moreover, miR-17 and miR-20a may play an important role in the development of EAE by affecting CD4 + T cells. The function of miR-17 in EAE mice has been previously reported [ 35 ], while the function of miR-20a remains unclear. We, therefore, chose miR-20a for further study.
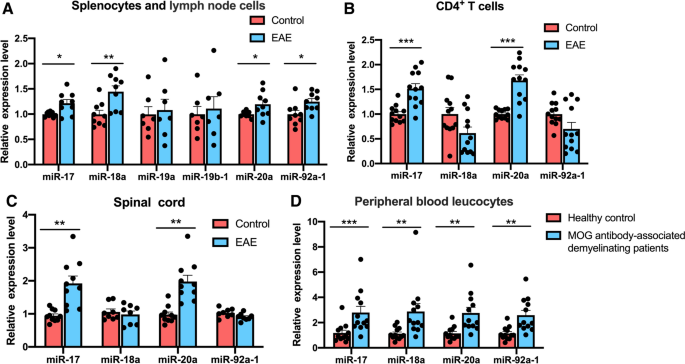
Different expression levels of miR-17–92 cluster in EAE mice and MOG antibody-associated demyelinating patients. A The expression levels of miR-17–92 cluster members in splenocytes and lymph node cells of EAE and control mice (n = 7–9). B The expression levels of miR-17–92 cluster members in isolated peripheral CD4 + T cells of EAE and control mice (n = 12). C The expression levels of miR-17–92 cluster members in spinal cord tissues of EAE and control mice (n = 8–10). D The expression levels of miR-17, miR-18a, miR-20a, and miR-92a-1 in the peripheral blood leucocytes of MOG antibody-associated demyelinating patients and healthy controls (HC) (n = 12). Data are shown as mean ± SEM. *p < 0.05, ** p < 0.01, *** p < 0.001 using unpaired Student’s t -test
miR-20a does not affect CD4 + T cell activation in EAE
We first explored whether miR-20a affects the activation of MOG-specific CD4 + T cells in EAE mice. To knock down the expression level of miR-20a, chemically modified antagomirs that are complementary to mature miR-20a were used. The knockdown efficiency of the miR-20a antagomirs is shown in Additional file 2 : Figure S1. Isolated CD4 + T cells from the spleens and lymph nodes of EAE mice were transfected with miR-20a or negative control (NC) antagomirs and stimulated with MOG 35-55 peptide for 3 days. Every day, activated CD4 + T cells defined as CD4 + CD62L low CD25 + or CD4 + CD69 + were analyzed by flow cytometry. The results showed that neither the percentage of CD4 + CD62L low CD25 + (Fig. 2 A, B) nor that of CD4 + CD69 + T cells (Fig. 2 C, D) was significantly different between the NC and miR-20a antagomir groups. The data indicated that miR-20a does not affect the activation of MOG-specific CD4 + T cells in EAE.
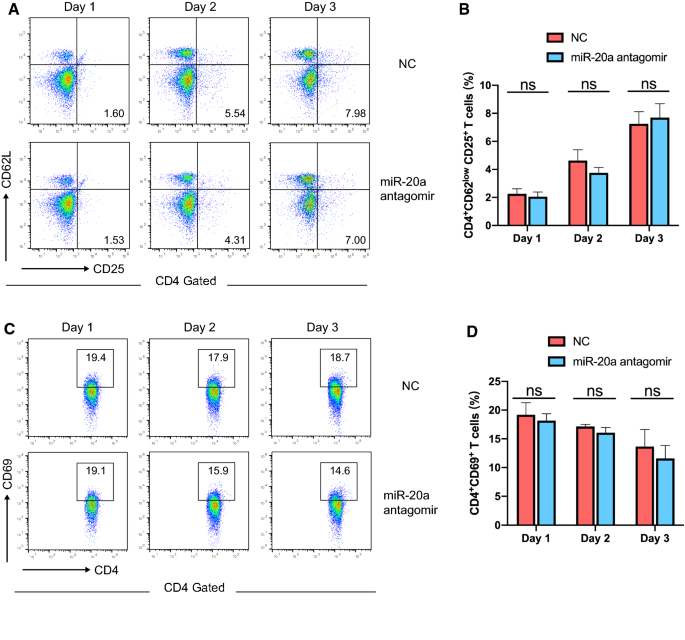
miR-20a does not affect the activation of MOG-specific CD4 + T cells in EAE mice. A , B The percentages of CD62L low CD25 + cells in the CD4 + gate, as measured by flow cytometry on Day 1, Day 2 and Day 3, separately. C , D The percentages of CD69 + cells in the CD4 + gate, as measured by flow cytometry on Day 1, Day 2 and Day 3, separately. The flow cytometry plots shown are representative of 3 independent experiments. Data are presented as mean ± SEM and ns represents no significant difference
miR-20a suppresses Treg differentiation in EAE
Since miR-20a does not affect the activation of CD4 + T cells in EAE, we next hypothesized that miR-20a is involved in the differentiation of CD4 + T cells. Th1 and Th17 cells are crucial effector cells in EAE initiation and progression [ 36 ], while Treg cells suppress excessive autoimmune responses [ 37 ]. We first examined the mRNA levels of characteristic cytokines and transcription factors of Treg, Th1, and Th17 cells in CD4 + T cells of EAE and control mice. The mRNA expression of Foxp3 was reduced in EAE mice compared to the control group (Fig. 3 A). Similarly, IL-10, the major anti-inflammatory cytokine secreted by Tregs, was also downregulated in EAE mice, though the expression of TGF-β1, another cytokine produced by Tregs, was comparable between the two groups (Fig. 3 B). It should be noted that although IFN-γ and IL-17A were upregulated in EAE mice, the mRNA expression levels of T-bet ( Tbx21 ) and RORγt ( Rorc ) were equivalent across the two groups (Fig. 3 A, B). Thus, we speculated that miR-20a may affect the differentiation of Treg cells. Naïve CD4 + T cells (CD4 + CD25 − CD44 − ) isolated from naïve mice were Treg-polarized in vitro for 4 days, and we found that the mRNA levels of both Foxp3 (Fig. 3 C) and IL-10 (Fig. 3 D) significantly increased in CD4 + T cells with miR-20a knockdown. We next polarized CD4 + T cells from EAE mice into Tregs with IL-2 and TGF-β1 under the MOG 35-55 stimulation. It was found that IL-2 and TGF-β1 successfully induced the differentiation of MOG-specific CD4 + T cells into Tregs under the MOG 35-55 stimulation (Fig. 3 E, F). Furthermore, the Treg differentiation was enhanced in the miR-20a knockdown group compared to the NC group. (Fig. 3 E, F). The results collectively indicated that miR-20a suppresses the differentiation of Treg cells while reducing the expression level of miR-20a promotes Treg differentiation.
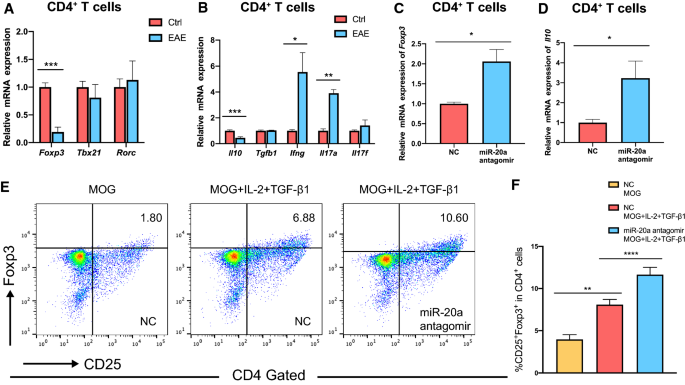
miR-20a suppresses Treg differentiation in EAE. A The mRNA levels of characteristic transcription factors of Treg ( Foxp3 ), Th1 ( Tbx21 ) and Th17 ( Rorc ) cells in CD4 + T cells of EAE and control mice. B The mRNA levels of characteristic cytokines of Treg ( Il-10 , Tgfb1 ), Th1 ( Ifng ) and Th17 ( Il-17a , Il-17f ) cells in CD4 + T cells of EAE and control mice. C The mRNA levels of Foxp3 in Treg-polarized CD4 + T cells with miR-20a or NC antagomirs transfected. D The mRNA levels of Il-10 in Treg-polarized CD4 + T cells with miR-20a or NC antagomirs transfected. E , F . The percentages of CD25 + Foxp3 + cells in CD4 + T cells of EAE mice after a 5-day MOG 35-55 stimulation and Treg polarization with miR-20a or NC antagomirs transfected. The flow cytometry plots shown are representative of 6 independent experiments. Data are shown as mean ± SEM. * p < 0.05, ** p < 0.01, **** p < 0.0001 using unpaired ( A – D ) and paired ( F ) Student’s t -test
Map3k9 is a potential target gene of miR-20a
We next investigated the mechanism by which miR-20a regulates the differentiation of Treg cells. We identified potential downstream gene targets of miR-20a using three online prediction tools based on the binding sites in the 3’ UTR, including TargetScan ( www.targetscan.org ), microT-CDS ( http://www.microrna.gr/webServer ) and miRDB ( www.mirdb.org ) (Fig. 4 A). Among all of the putative gene targets, 532 overlapping targets of the three programs were functional enrichment analyzed on GO and KEGG pathway databases by the WebGestalt online tool ( http://www.webgestalt.org ) (Fig. 4 B, C). We selected some potential gene targets that are functionally involved in inflammatory responses and tested their mRNA levels in splenocytes and lymph node cells of EAE and control mice. The expression of Map3k9 (mitogen-activated protein kinase kinase kinase 9) was downregulated in EAE mice (Fig. 4 D), which makes Map3k9 a potential gene target of miR-20a. Tgfbr2 (transforming growth factor beta receptor 2) was also downregulated in EAE mice; however, we next found that Tgfbr2 was upregulated in CD4 + T cells of EAE mice, which refuted the speculation that Tgfbr2 was a miR-20a target gene (data not shown).
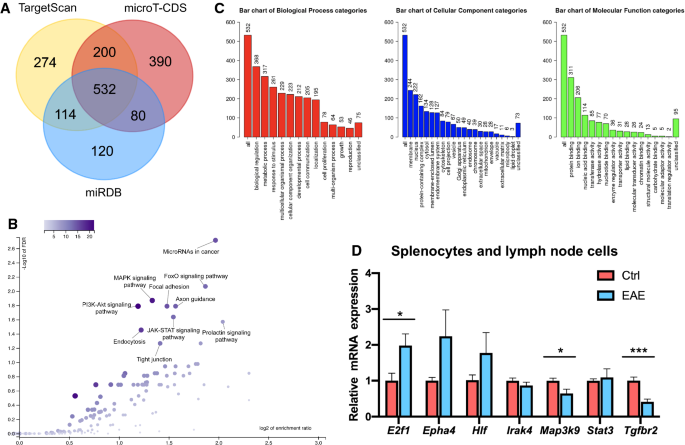
Prediction and initial validation of the target genes of miR-20a. A The potential target genes of miR-20a predicted by TargetScan, microT-CDS, and miRDB. 532 overlapping targets predicted by the three online programs were then functional enrichment analyzed. B a GO slim summary for the 532 target genes annotated by the online WebGestalt tool. C Functional annotation of the 532 overlapping genes based on KEGG pathway Database by the online WebGestalt tool. D The mRNA expression levels of 7 selected potential target genes in EAE and control mice (n = 6–9). Data are shown as mean ± SEM. * p < 0.05, *** p < 0.001 using unpaired Student’s t -test
Expression of Map3k9 is influenced by miR-20a
Since Map3k9 was downregulated in EAE mice when miR-20a was upregulated, we next investigated the correlation between the expression of Map3k9 and miR-20a. The mRNA level of Map3k9 was decreased in CD4 + T cells in EAE mice (Fig. 5 A). Furthermore, the mRNA and protein levels of Map3k9 were also decreased in the spinal cords of EAE mice (Fig. 5 B–D). In addition, by linear regression analysis, a significant negative correlation between the mRNA levels of miR-20a and Map3k9 was shown in both CD4 + T cells and spinal cord tissues from EAE and control mice (Fig. 5 E, F). We finally validated this result in patients and found that the mRNA level of Map3k9 was decreased in peripheral blood leukocytes from patients with MOG antibody-associated demyelinating disease compared to healthy controls (Fig. 5 G). Taken together, these results indicate that the expression of Map3k9 is influenced by that of miR-20a.
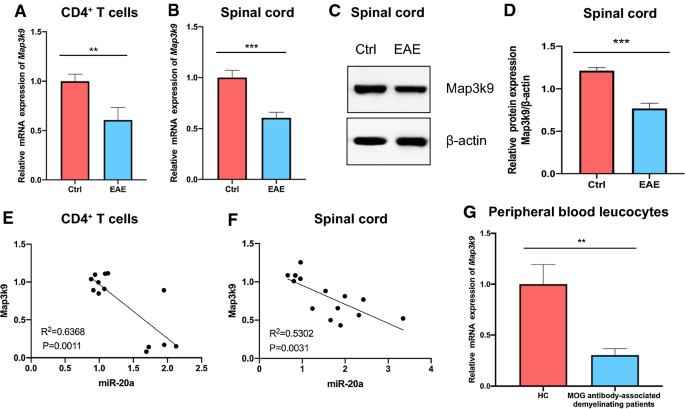
The expression of Map3k9 is influenced by miR-20a. A The mRNA expression level of Map3k9 in CD4 + T cells from EAE and control mice. B The mRNA expression level of Map3k9 in spinal cord tissues from EAE and control mice. C , D . The protein expression level of Map3k9 in spinal cord tissues from EAE and control mice tested by western blot. E Linear regression analysis of the correlation between the expression of miR-20a and Map3k9 in CD4 + T cells from EAE and control mice. F Linear regression analysis of the correlation between the expression of miR-20a and Map3k9 in spinal cord tissues from EAE and control mice. G The mRNA level of Map3k9 in the peripheral blood leucocytes of MOG antibody-associated demyelinating patients and HCs. Data are shown as mean ± SEM. ** p < 0.01, *** p < 0.001 using unpaired Student’s t -test
Map3k9 is a target gene of miR-20a to suppress Treg differentiation in EAE
To determine whether Map3k9 is the target gene for miR-20a to suppress Treg differentiation in EAE, we first knocked down the expression of miR-20a in CD4 + T cells and observed a significantly increased expression of Map3k9 (Fig. 6 A). According to the results of target gene prediction, a putative binding site for miR-20a in the 3’ untranslated region (UTR) of Map3k9 was identified. We next performed luciferase reporter assays to detect the binding of miR-20a and the 3’ UTR of Map3k9 (Fig. 6 B). The results showed that miR-20a specifically suppressed the luciferase activity of the reporter containing the wild-type 3’ UTR of Map3k9 while the reporter containing a mutant or NC 3’ UTR was not affected, indicating effective and specific binding of miR-20a to the predicted binding site in Map3k9 (Fig. 6 C). At this point, we have demonstrated that Map3k9 is a target gene of miR-20a.
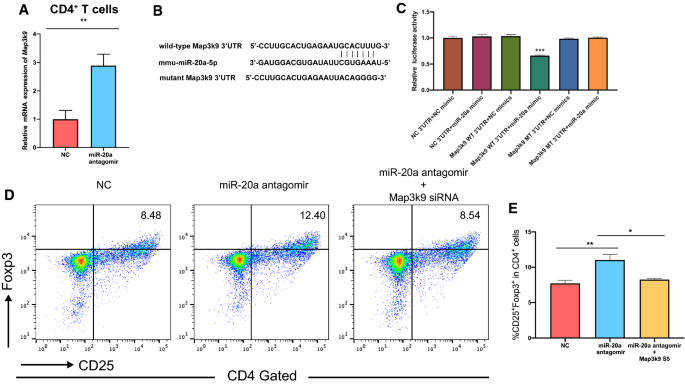
Map3k9 is the target gene of miR-20a to suppress Treg differentiation in EAE. A The expression of Map3k9 was upregulated in CD4 + T cells with miR-20a knockdown. B Sequences of WT and mutant 3’ UTR of Map3k9 and miR-20a mimics used in the luciferase assays. C Relative luciferase activity of the reporter carrying the WT or mutant Map3k9 3’ UTR or NC 3’ UTR co-transfected with miR-20a mimics or NC mimics into HEK-293 cells. The activity of Renilla luciferase was used to eliminate variances between groups. D , E Flow cytometric analysis of the CD4 + T cells transfected with NC or miR-20a antagomirs, or miR-20a antagomirs + Map3k9 siRNA after stimulated with MOG 35-55 peptide in Treg-polarizing condition for 5 days. The percentages of CD25 + Foxp3 + cells are shown in the CD4 + gate. The flow cytometry plots shown are representative of 4 independent experiments. Data are shown as mean ± SEM. * p < 0.05, ** p < 0.01, *** p < 0.001 using unpaired ( A , C ) and paired ( E ) Student’s t -test
We finally explored whether miR-20a inhibits the differentiation of Tregs in EAE mice by reducing the expression of Map3k9. To this end, isolated CD4 + T cells were transfected with antagomirs or were cotransfected with miR-20a antagomirs and Map3k9 siRNA (The knockdown efficiency of the siRNA is shown in Additional file 3 : Figure S2). The cells were polarized into Tregs under MOG 35-55 stimulation as mentioned above. The results showed that CD4 + T cells transfected with the miR-20a antagomirs alone displayed an enhanced capacity to differentiate into Treg cells, which was consistent with the previous results (Fig. 6 D, E). However, the results did not show any difference in Treg differentiation between the CD4 + T cells transfected with miR-20a antagomirs + Map3k9 siRNA and the NC antagomirs (Fig. 6 D, E). The findings suggested that miR-20a suppresses differentiation of MOG-specific CD4 + T cells into Tregs in EAE mice by reducing the expression of Map3k9.
Collectively, our results suggest that Map3k9 is a functional target of miR-20a to suppress Treg differentiation in EAE.
miR-20a antagomir treatment alleviates the severity of EAE in vivo
As described above, we demonstrated that miR-20a suppresses Treg differentiation of MOG-specific CD4 + T cells in EAE mice. We next explored whether miR-20a knockdown in EAE mice could alleviate the severity of EAE in vivo. We injected EAE mice intravenously with miR-20a or the NC antagomirs for 3 consecutive days at the onset of the disease and observed the clinical scores until the remission stage. The highest clinical score for mice injected with miR-20a antagomirs was 2.5 compared to 3.5 in mice injected with the NC antagomirs. In addition, as shown in Fig. 7 , the average area under the scoring curve of the miR-20a antagomir group throughout the observation period was smaller than that of the NC group. For the individual days, the scores for the miR-20a antagomir group on Day 18 and Day 19 postimmunization were lower than those for the NC group. All of the above results indicated that miR-20a knockdown with antagomirs attenuates the clinical symptoms of EAE.
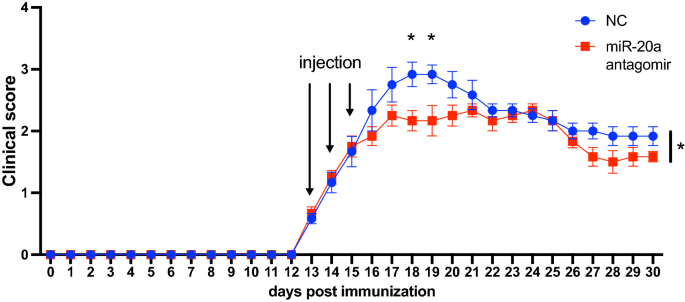
miR-20a antagomir treatment alleviates the clinical symptoms of EAE mice. The EAE mice received consecutive intravenous injections of antagomirs at the onset of the disease for 3 days and the observation period was 30 days post immunization (n = 6 per group). Data are shown as mean ± SEM. * p < 0.05 using unpaired Student’s t -test for comparing the area under curve (AUC) and Mann–Whitney test for comparing clinical scores for individual days
We also performed the pathological analysis of spinal cord sections from EAE mice by hematoxylin and eosin (H&E) staining and Luxol fast blue staining. The amount of infiltrating inflammatory cells in the miR-20a antagomir group was less than that in the NC group (Fig. 8 A–E). In addition, the percentage of demyelinated area to total white matter area (demyelinated area %) was lower in the miR-20a antagomir group than that in the NC group (Fig. 9 A–E). Furthermore, by analyzing the CD4 + T cells from the two groups at the remission stage of the disease with flow cytometry, we observed that the percentages of IFN-γ + Th1 cells and IL-17A + Th17 cells in the CD4 + gate were similar between the miR-20a antagomir and NC groups (Fig. 10 A–C), while the percentage of CD25 + Foxp3 + Treg cells in the miR-20a antagomir group was higher than that in the NC group (Fig. 10 D, E). Collectively, the results showed that miR-20a knockdown with antagomirs alleviates the clinical symptoms of EAE, decreases inflammatory infiltration, reduces myelin damage, and promotes the differentiation of Treg cells in EAE mice. These findings suggest a potential therapeutic role of miR-20a antagomirs in the treatment of EAE and CNS inflammatory demyelinating diseases.
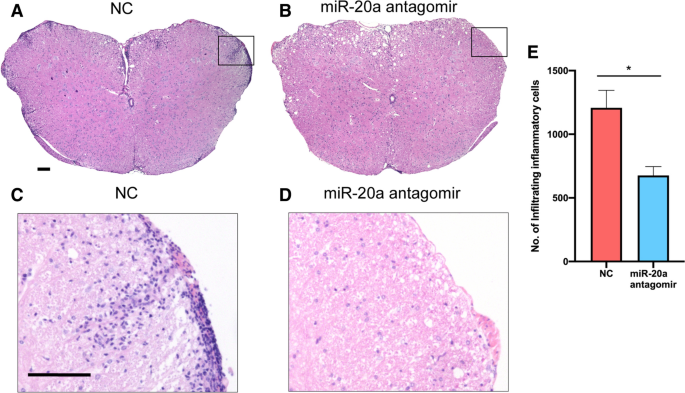
miR-20a antagomir treatment reduces the infiltrating inflammatory cells in spinal cords of EAE mice. A Representative H&E staining of complete spinal cord sections from NC group (taken at × 10 magnification). B Representative H&E staining of complete spinal cord sections from miR-20a antagomir group (taken at × 10 magnification). C Representative H&E staining of partial spinal cord sections from NC group (taken at × 20 magnification). D Representative H&E staining of partial spinal cord sections from miR-20a antagomir group (taken at × 20 magnification). E Quantification of the infiltrating inflammatory cells in the two groups. Scale bars correspond to 100 μm. Data are shown as mean ± SEM. * p < 0.05 using unpaired Student’s t -test
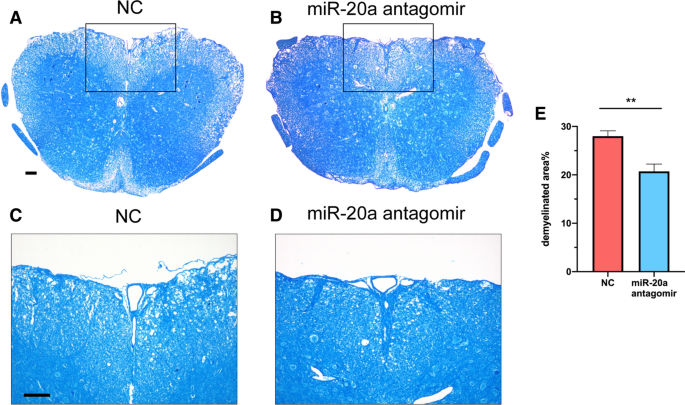
miR-20a antagomir treatment decreases demyelinated areas in spinal cords of EAE mice. A Representative Luxol fast blue staining of complete spinal cord sections from NC group (taken at × 10 magnification). B Representative Luxol fast blue staining of complete spinal cord sections from miR-20a antagomir group (taken at × 10 magnification). C Representative Luxol fast blue staining of partial spinal cord sections from NC group (taken at × 20 magnification). D Representative Luxol fast blue staining of partial spinal cord sections from miR-20a antagomir group (taken at × 20 magnification). E Quantification of the percentage of demyelinated area to total white matter area in the two groups. Scale bars correspond to 100 μm. The demyelinated areas and total white matter areas were calculated with ImageJ software. Data are shown as mean ± SEM. ** p < 0.01 using unpaired Student’s t -test

miR-20a antagomir treatment promotes Treg differentiation in EAE mice. A – C Flow cytometric analysis of IFN-γ + and IL-17A + cells in CD4 + T cells of miR-20a antagomir and control group. D , E Flow cytometric analysis of CD25 + Foxp3 + cells in CD4 + T cells of miR-20a antagomir and control group. The flow cytometry plots shown are representative of 4 independent experiments. Data are shown as mean ± SEM. * p < 0.05 using unpaired Student’s t -test
The miR-17-92 cluster was first identified because of its oncogenic effects in a variety of tumors, such as leukemia, lymphoma, and cancers derived from the colon, breast, ovary, and pancreas [ 22 , 24 ]. In recent years, an increasing number of studies have shown the involvement of miR-17-92 in autoimmune diseases. It is found that miR-17-92 is a critical regulator of follicular helper T (Tfh) cells differentiation in an ovalbumin (OVA)-induced model of autoimmunity [ 38 ]. miR-17-92 also plays an essential role in the activation, proliferation, survival, and differentiation of CD4 + T cells in graft-versus-host disease (GVHD) [ 27 , 28 ]. In addition, members of the miR-17–92 cluster were upregulated in CD4 + T cells from patients with systemic lupus erythematosus (SLE) [ 39 ], and miR-17 affects TNF-α signaling in rheumatoid arthritis [ 40 ]. Members of the miR-17-92 cluster are also dysregulated in CNS inflammatory demyelinating diseases, and some of them are restored to normal levels after medical treatment or during remission [ 30 , 32 , 33 , 34 , 41 , 42 , 43 , 44 ]. The findings in some studies are contradictory, which may be attributed to the different clinical stages of the patients; however, miR-17-92 cluster is still recognized as an important regulator of CNS inflammatory demyelinating diseases. Nevertheless, the role and mechanism of miR-17-92 cluster in CNS inflammatory demyelinating diseases are still unclear and remain to be further explored.
In this study, we observed the upregulation of miR-20a in splenocytes and lymph node cells from EAE mice and peripheral blood leukocytes from patients with MOG antibody-associated demyelinating diseases and further found that miR-20a was upregulated in CD4 + T cells and spinal cord tissues from EAE mice. EAE is mediated mainly by CD4 + T cells in which Th1 and Th17 cells are major pathogenic cells while Treg cells play an important role in suppressing inflammatory responses. We speculated that miR-20a may involve in the development of EAE by targeting CD4 + T cells. In our study, no evidence showed that miR-20a affects the activation of MOG-specific CD4 + T cells, we then found for the first time that miR-20a knockdown promotes the differentiation of MOG-specific CD4 + T cells into Tregs, revealing the role of miR-20a in suppressing Treg differentiation in EAE. Jin et al. found that protectin DX increases the proportion of Tregs in a collagen-induced arthritis model by inhibiting NLRP3 inflammasome via miR-20a [ 45 ], which appears to be contrary to the findings in our study. However, the animal models used in these two studies are quite different and the important influencing factor in Jin’s study, protectin DX treatment, was not involved in our study, which makes contrasting results in the two studies possible.
In addition, in this study, we observed that miR-20a knockdown using antagomirs in EAE mice in vivo did not affect the proportion of IFN-γ + Th1 and IL-17A + Th17 cells. However, Chang et al. found that miR-20a reduces the proportion of IL-17 + cells in CD4 + T cells of patients with Vogt-Koyanagi-Harada disease by targeting OSM and CCL1 [ 46 ]. We speculate that the differences between their findings and ours are due to the following reasons. First, the diseases investigated in the two studies are different, which leads to completely different pathological states of the CD4 + T cells. Second, the results in Chang’s study were obtained through in vitro experiments, whereas our findings were obtained through in vivo experiments, which causes the different results in the two studies.
We further investigated the target gene of miR-20a and demonstrated that Map3k9 is a functional target gene of miR-20a to suppress Treg differentiation in EAE through luciferase assays and functional validation. Map3k9, also known as mixed-lineage kinase 1 (MLK1), is an important upstream component in the MAPK pathway as a mitogen-activated protein kinase kinase kinase to activate the c-Jun amino-terminal kinase (JNK), p38, and extracellular-signal regulated kinase (ERK) pathways [ 47 , 48 , 49 ]. The MAPK signaling pathway plays an important role in a variety of cellular processes, including proliferation, differentiation, survival, apoptosis, and immune response [ 50 ]. In previous studies, downstream components of the MAPK signaling pathway have been found to influence multiple activities of Tregs. Bao et al. demonstrated that JNK increases the levels of Foxp3 protein in CD4 + CD25 + Treg cells by binding to its promoters [ 51 ]. Lu et al. found that bone morphogenetic protein (BMP)-2/4 promotes the differentiation of Tregs induced by TGF-β through phosphorylated ERK and JNK [ 52 ]. In addition, another study showed that inhibiting the activation of p38 abrogates the proliferation and Foxp3 expression in Tregs induced by tumor necrosis factor (TNF) [ 53 ]. Furthermore, it has been revealed that the regulatory function of iTregs is associated with enhanced p38 activity [ 54 ]. In this study, we found for the first time that an upstream component of the MAPK signaling pathway, Map3k9, enhances the differentiation of Tregs as a direct target of a miRNA, which further extends the findings on the MAPK signaling pathway.
There are still some limitations in this study. First, the optimal dose, timing, and delivery mode of administration of miR-20a antagomirs to treat EAE mice were not investigated in detail. Considering that miR-20a antagomirs are expected to be a treatment for EAE in the future, the issues described above require more intensive exploration. Second, although the results of patients with MOG antibody-associated demyelinating diseases were consistent with those of EAE mice, it is still inconclusive whether the EAE model perfectly simulates MOG antibody-associated demyelinating diseases. In the following studies, we need to isolate CD4 + T cells from patients to validate the results of EAE mice. In addition, we did not validate the results of EAE mice in MS patients, which is another limitation. We will validate the results above in MS patients and compare the results of EAE mice, MS patients, and MOG antibody-associated demyelinating diseases patients in the later studies.
In this study, we found for the first time that miR-20a suppresses the Treg differentiation of MOG-specific CD4 + T cells in EAE by reducing the expression of Map3k9. In addition, our findings indicate the potential role of miR-20a antagomirs in therapies for EAE and CNS inflammatory demyelinating disease. Since the therapeutic effects of a growing number of miRNAs have been verified in clinical trials [ 55 ], we expect miR-20a to play a role in the clinical therapies of CNS inflammatory demyelinating diseases in the future.
In this study, we demonstrated that miR-20a suppresses the differentiation of MOG-specific CD4 + T cells into Tregs in EAE mice by decreasing the expression of Map3k9. Administration of miR-20a antagomirs to EAE mice reduces the severity of the disease, suggesting a potential role of miR-20a in therapies for EAE and CNS inflammatory demyelinating diseases.
Availability of data and materials
All data are available in the manuscript or upon request to the authors.
Abbreviations
Central nervous system
- Experimental autoimmune encephalomyelitis
Untranslated region
Multiple sclerosis
Neuromyelitis optica spectrum disorder
Myelin oligodendrocyte glycoprotein
- Regulatory T cell
Induced regulatory T cell
Follicular helper T cell
Interferon-γ
Interleukin
Granulocyte–macrophage colony-stimulating factor
Transforming growth factor-β1
Forkhead box P3
RAR-related orphan receptor gamma-t
Gene Ontology
Kyoto Encyclopedia of Genes and Genomes
Mitogen-activated protein kinase kinase kinase 9
Transforming growth factor beta receptor 2
Complete Freund’s adjuvant
Pertussis toxin
Magnetic bead cell sorting
Small interfering RNA
Negative control
N-2-Hydroxyethylpiperazine-N-2-Ethane Sulfonic Acid
Radioimmunoprecipitation assay
Ethylenediamine tetraacetic acid
Sodium dodecyl sulfate
Polyacrylamide gel
Polyvinylidene fluoride
Bovine serum albumin
Hematoxylin and eosin
Graft-versus-host disease
Systemic lupus erythematosus
Mixed-lineage kinase 1
C-Jun amino-terminal kinase
Extracellular-signal regulated kinase
Tumor necrosis factor
Bone morphogenetic protein
Barnett MH, Mathey E, Kiernan MC, Pollard JD. Axonal damage in central and peripheral nervous system inflammatory demyelinating diseases: common and divergent pathways of tissue damage. Curr Opin Neurol. 2016;29(3):213–21.
Article CAS PubMed Google Scholar
Storch M, Lassmann H. Pathology and pathogenesis of demyelinating diseases. Curr Opin Neurol. 1997;10(3):186–92.
Takai Y, Misu T, Kaneko K, Chihara N, Narikawa K, Tsuchida S, et al. Myelin oligodendrocyte glycoprotein antibody-associated disease: an immunopathological study. Brain. 2020;143(5):1431–46.
Article PubMed Google Scholar
Ochi H, Fujihara K. Demyelinating diseases in Asia. Curr Opin Neurol. 2016;29(3):222–8.
Steinman L. Immunology of relapse and remission in multiple sclerosis. Annu Rev Immunol. 2014;32:257–81.
Hemmer B, Kerschensteiner M, Korn T. Role of the innate and adaptive immune responses in the course of multiple sclerosis. Lancet Neurol. 2015;14(4):406–19.
Thompson AJ, Baranzini SE, Geurts J, Hemmer B, Ciccarelli O. Multiple sclerosis. Lancet. 2018;391(10130):1622–36.
Chu F, Shi M, Zheng C, Shen D, Zhu J, Zheng X, et al. The roles of macrophages and microglia in multiple sclerosis and experimental autoimmune encephalomyelitis. J Neuroimmunol. 2018;318:1–7.
Glatigny S, Bettelli E. Experimental Autoimmune Encephalomyelitis (EAE) as animal models of multiple sclerosis (MS). Cold Spring Harb Perspect Med. 2018;8(11):a028977.
Article CAS PubMed PubMed Central Google Scholar
Simmons SB, Pierson ER, Lee SY, Goverman JM. Modeling the heterogeneity of multiple sclerosis in animals. Trends Immunol. 2013;34(8):410–22.
Hoftberger R, Guo Y, Flanagan EP, Lopez-Chiriboga AS, Endmayr V, Hochmeister S, et al. The pathology of central nervous system inflammatory demyelinating disease accompanying myelin oligodendrocyte glycoprotein autoantibody. Acta Neuropathol. 2020;139(5):875–92.
Article PubMed PubMed Central CAS Google Scholar
Lassmann H. The changing concepts in the neuropathology of acquired demyelinating central nervous system disorders. Curr Opin Neurol. 2019;32(3):313–9.
Kunkl M, Frascolla S, Amormino C, Volpe E, Tuosto L. T helper cells: the modulators of inflammation in multiple sclerosis. Cells. 2020;9(2):482.
Article CAS PubMed Central Google Scholar
Segal BM. The diversity of encephalitogenic CD4+ T cells in multiple sclerosis and its animal models. J Clin Med. 2019;8(1):120.
Yasuda K, Takeuchi Y, Hirota K. The pathogenicity of Th17 cells in autoimmune diseases. Semin Immunopathol. 2019;41(3):283–97.
Cheng Y, Sun L, Xie Z, Fan X, Cao Q, Han J, et al. Diversity of immune cell types in multiple sclerosis and its animal model: Pathological and therapeutic implications. J Neurosci Res. 2017;95(10):1973–83.
Pierson E, Simmons SB, Castelli L, Goverman JM. Mechanisms regulating regional localization of inflammation during CNS autoimmunity. Immunol Rev. 2012;248(1):205–15.
Article PubMed PubMed Central Google Scholar
Broux B, Markovic-Plese S, Stinissen P, Hellings N. Pathogenic features of CD4+CD28- T cells in immune disorders. Trends Mol Med. 2012;18(8):446–53.
Kleinewietfeld M, Hafler DA. Regulatory T cells in autoimmune neuroinflammation. Immunol Rev. 2014;259(1):231–44.
Jonas S, Izaurralde E. Towards a molecular understanding of microRNA-mediated gene silencing. Nat Rev Genet. 2015;16(7):421–33.
Rupaimoole R, Slack FJ. MicroRNA therapeutics: towards a new era for the management of cancer and other diseases. Nat Rev Drug Discov. 2017;16(3):203–22.
Mendell JT. miRiad roles for the miR-17-92 cluster in development and disease. Cell. 2008;133(2):217–22.
Olive V, Li Q, He L. mir-17-92: a polycistronic oncomir with pleiotropic functions. Immunol Rev. 2013;253(1):158–66.
Mogilyansky E, Rigoutsos I. The miR-17/92 cluster: a comprehensive update on its genomics, genetics, functions and increasingly important and numerous roles in health and disease. Cell Death Differ. 2013;20(12):1603–14.
Jiang S, Li C, Olive V, Lykken E, Feng F, Sevilla J, et al. Molecular dissection of the miR-17-92 cluster’s critical dual roles in promoting Th1 responses and preventing inducible Treg differentiation. Blood. 2011;118(20):5487–97.
Xiao C, Srinivasan L, Calado DP, Patterson HC, Zhang B, Wang J, et al. Lymphoproliferative disease and autoimmunity in mice with increased miR-17-92 expression in lymphocytes. Nat Immunol. 2008;9(4):405–14.
Wu Y, Schutt S, Paz K, Zhang M, Flynn RP, Bastian D, et al. MicroRNA-17-92 is required for T-cell and B-cell pathogenicity in chronic graft-versus-host disease in mice. Blood. 2018;131(17):1974–86.
Wu Y, Heinrichs J, Bastian D, Fu J, Nguyen H, Schutt S, et al. MicroRNA-17-92 controls T-cell responses in graft-versus-host disease and leukemia relapse in mice. Blood. 2015;126(11):1314–23.
Ingwersen J, Menge T, Wingerath B, Kaya D, Graf J, Prozorovski T, et al. Natalizumab restores aberrant miRNA expression profile in multiple sclerosis and reveals a critical role for miR-20b. Ann Clin Transl Neurol. 2015;2(1):43–55.
Meira M, Sievers C, Hoffmann F, Rasenack M, Kuhle J, Derfuss T, et al. Unraveling natalizumab effects on deregulated miR-17 expression in CD4+ T cells of patients with relapsing-remitting multiple sclerosis. J Immunol Res. 2014;214:897249.
Google Scholar
Keller A, Leidinger P, Steinmeyer F, Stahler C, Franke A, Hemmrich-Stanisak G, et al. Comprehensive analysis of microRNA profiles in multiple sclerosis including next-generation sequencing. Mult Scler. 2014;20(3):295–303.
Lindberg RL, Hoffmann F, Mehling M, Kuhle J, Kappos L. Altered expression of miR-17-5p in CD4+ lymphocytes of relapsing-remitting multiple sclerosis patients. Eur J Immunol. 2010;40(3):888–98.
Gandhi R, Healy B, Gholipour T, Egorova S, Musallam A, Hussain MS, et al. Circulating microRNAs as biomarkers for disease staging in multiple sclerosis. Ann Neurol. 2013;73(6):729–40.
Dolati S, Aghebati-Maleki L, Ahmadi M, Marofi F, Babaloo Z, Ayramloo H, et al. Nanocurcumin restores aberrant miRNA expression profile in multiple sclerosis, randomized, double-blind, placebo-controlled trial. J Cell Physiol. 2018;233(7):5222–30.
Liu SQ, Jiang S, Li C, Zhang B, Li QJ. miR-17-92 cluster targets phosphatase and tensin homology and Ikaros Family Zinc Finger 4 to promote TH17-mediated inflammation. J Biol Chem. 2014;289(18):12446–56.
Jager A, Dardalhon V, Sobel RA, Bettelli E, Kuchroo VK. Th1, Th17, and Th9 effector cells induce experimental autoimmune encephalomyelitis with different pathological phenotypes. J Immunol. 2009;183(11):7169–77.
Article PubMed CAS Google Scholar
Lee PW, Severin ME, Lovett-Racke AE. TGF-β regulation of encephalitogenic and regulatory T cells in multiple sclerosis. Eur J Immunol. 2017;47(3):446–53.
Kang SG, Liu WH, Lu P, Jin HY, Lim HW, Shepherd J, et al. MicroRNAs of the miR-17 approximately 92 family are critical regulators of T (FH) differentiation. Nat Immunol. 2013;14(8):849–57.
Qin HH, Zhu XH, Liang J, Wu JF, Yang YS, Xu JH. The expression and significance of miR-17-92 cluster miRs in CD4 (+) T cells from patients with systemic lupus erythernatosus. Clin Exp Rheumatol. 2013;31(3):472–3.
PubMed Google Scholar
Akhtar N, Singh AK, Ahmed S. MicroRNA-17 Suppresses TNF-alpha Signaling by Interfering with TRAF2 and cIAP2 Association in Rheumatoid Arthritis Synovial Fibroblasts. J Immunol. 2016;197(6):2219–28.
Cox MB, Cairns MJ, Gandhi KS, Carroll AP, Moscovis S, Stewart GJ, et al. MicroRNAs miR-17 and miR-20a inhibit T cell activation genes and are under-expressed in MS whole blood. PLoS ONE. 2010;5(8):e12132.
Keller A, Leidinger P, Steinmeyer F, Stähler C, Franke A, Hemmrich-Stanisak G, et al. Comprehensive analysis of microRNA profiles in multiple sclerosis including next-generation sequencing. Mult Scler. 2014;20(3):295–303.
Ehtesham N, Khorvash F, Kheirollahi M. miR-145 and miR20a-5p potentially mediate pleiotropic effects of interferon-beta through mitogen-activated protein kinase signaling pathway in multiple sclerosis patients. J Mol Neurosci. 2017;61(1):16–24.
Ghadiri N, Emamnia N, Ganjalikhani-Hakemi M, Ghaedi K, Etemadifar M, Salehi M, et al. Analysis of the expression of mir-34a, mir-199a, mir-30c and mir-19a in peripheral blood CD4+T lymphocytes of relapsing-remitting multiple sclerosis patients. Gene. 2018;659:109–17.
Jin S, Sun S, Ling H, Ma J, Zhang X, Xie Z, et al. Protectin DX restores Treg/Th17 cell balance in rheumatoid arthritis by inhibiting NLRP3 inflammasome via miR-20a. Cell Death Dis. 2021;12(3):280.
Chang R, Yi S, Tan X, Huang Y, Wang Q, Su G, et al. MicroRNA-20a-5p suppresses IL-17 production by targeting OSM and CCL1 in patients with Vogt-Koyanagi-Harada disease. Br J Ophthalmol. 2018;102(2):282–90.
Durkin JT, Holskin BP, Kopec KK, Reed MS, Spais CM, Steffy BM, et al. Phosphoregulation of mixed-lineage kinase 1 activity by multiple phosphorylation in the activation loop. Biochemistry. 2004;43(51):16348–55.
Gallo KA, Johnson GL. Mixed-lineage kinase control of JNK and p38 MAPK pathways. Nat Rev Mol Cell Biol. 2002;3(9):663–72.
Marusiak AA, Edwards ZC, Hugo W, Trotter EW, Girotti MR, Stephenson NL, et al. Mixed lineage kinases activate MEK independently of RAF to mediate resistance to RAF inhibitors. Nat Commun. 2014;5:3901.
Kim EK, Choi EJ. Compromised MAPK signaling in human diseases: an update. Arch Toxicol. 2015;89(6):867–82.
Bao R, Hou J, Li Y, Bian J, Deng X, Zhu X, et al. Adenosine promotes Foxp3 expression in Treg cells in sepsis model by activating JNK/AP-1 pathway. Am J Transl Res. 2016;8(5):2284–92.
CAS PubMed PubMed Central Google Scholar
Lu L, Ma J, Wang X, Wang J, Zhang F, Yu J, et al. Synergistic effect of TGF-beta superfamily members on the induction of Foxp3+ Treg. Eur J Immunol. 2010;40(1):142–52.
He T, Liu S, Chen S, Ye J, Wu X, Bian Z, et al. The p38 MAPK inhibitor SB203580 abrogates tumor necrosis factor-induced proliferative expansion of mouse CD4 (+)Foxp3 (+) regulatory T cells. Front Immunol. 2018;9:1556.
Adler HS, Kubsch S, Graulich E, Ludwig S, Knop J, Steinbrink K. Activation of MAP kinase p38 is critical for the cell-cycle-controlled suppressor function of regulatory T cells. Blood. 2007;109(10):4351–9.
Sun P, Liu DZ, Jickling GC, Sharp FR, Yin KJ. MicroRNA-based therapeutics in central nervous system injuries. J Cereb Blood Flow Metab. 2018;38(7):1125–48.
Download references
Acknowledgements
We give our sincere gratitude to the patients who participated in this study and the reviewers for their valuable suggestions.
This research was supported by grants from the National Natural Science Foundation of China (81771295, 82071341, 81801195), the Special Construction Fund for Integrating Chinese and Western Medicine in General Hospitals (ZHYY-ZXJHZX-1–201701), and Innovative research team of high-level local universities in Shanghai and Shanghai Sailing program (18YF1413100).
Author information
Authors and affiliations.
Department of Neurology, Renji Hospital, Shanghai Jiaotong University School of Medicine, 160 Pujian Road, Shanghai, 200127, China
Yishu Wang, Chong Xie, Yaying Song, Weiwei Xiang, Jing Peng, Lu Han, Jie Ding & Yangtai Guan
You can also search for this author in PubMed Google Scholar
Contributions
YW designed the experiments, performed the cellular experiments and drafted the manuscript; CX and YS coordinated the study and edited the manuscript; WX and JP performed the animal experiments; LH and JD provided technical support; YG conceived the study, edited the manuscript, and administered the project. All authors read and approved the final manuscript.
Corresponding author
Correspondence to Yangtai Guan .
Ethics declarations
Ethics approval and consent to participate.
All animal experiments were approved by the Animal Ethics and Welfare Committee of Renji Hospital affiliated to Shanghai Jiao Tong University School of Medicine. All experiments involving human blood samples were approved by the Ethics Committee of the Renji Hospital affiliated to Shanghai Jiao Tong University School of Medicine.
Consent for publication
Not applicable.
Competing interests
The authors declare that they have no competing interests.
Additional information
Publisher's note.
Springer Nature remains neutral with regard to jurisdictional claims in published maps and institutional affiliations.
Supplementary Information
Additional file 1.
: Table S1. The primers used in the study.
Additional file 2
: Figure S1. The knockdown efficiency of the miR-20a antagomirs.
Additional file 3
: Figure S2. The knockdown efficiency of the Map3k9 siRNA.
Rights and permissions
Open Access This article is licensed under a Creative Commons Attribution 4.0 International License, which permits use, sharing, adaptation, distribution and reproduction in any medium or format, as long as you give appropriate credit to the original author(s) and the source, provide a link to the Creative Commons licence, and indicate if changes were made. The images or other third party material in this article are included in the article's Creative Commons licence, unless indicated otherwise in a credit line to the material. If material is not included in the article's Creative Commons licence and your intended use is not permitted by statutory regulation or exceeds the permitted use, you will need to obtain permission directly from the copyright holder. To view a copy of this licence, visit http://creativecommons.org/licenses/by/4.0/ . The Creative Commons Public Domain Dedication waiver ( http://creativecommons.org/publicdomain/zero/1.0/ ) applies to the data made available in this article, unless otherwise stated in a credit line to the data.
Reprints and permissions
About this article
Cite this article.
Wang, Y., Xie, C., Song, Y. et al. miR-20a suppresses Treg differentiation by targeting Map3k9 in experimental autoimmune encephalomyelitis. J Transl Med 19 , 223 (2021). https://doi.org/10.1186/s12967-021-02893-4
Download citation
Received : 13 April 2021
Accepted : 17 May 2021
Published : 26 May 2021
DOI : https://doi.org/10.1186/s12967-021-02893-4
Share this article
Anyone you share the following link with will be able to read this content:
Sorry, a shareable link is not currently available for this article.
Provided by the Springer Nature SharedIt content-sharing initiative
- Inflammatory demyelinating diseases of the central nervous system
Journal of Translational Medicine
ISSN: 1479-5876
- Submission enquiries: Access here and click Contact Us
- General enquiries: [email protected]
Novel disease-modifying anti-rheumatic drug iguratimod suppresses chronic experimental autoimmune encephalomyelitis by down-regulating activation of macrophages/microglia through an NF-κB pathway
Affiliations.
- 1 Department of Neurology, Neurological Institute, Graduate School of Medical Sciences, Kyushu University, Fukuoka, 812-8582, Japan.
- 2 Department of Neurology, Neurological Institute, Graduate School of Medical Sciences, Kyushu University, Fukuoka, 812-8582, Japan. [email protected].
- 3 Department of Geriatric Medicine and Neurology, Ehime University Graduate School of Medicine, Matsuyama, 791-0295, Japan.
- 4 Department of Neurology, Neurological Institute, Graduate School of Medical Sciences, Kyushu University, Fukuoka, 812-8582, Japan. [email protected].
- PMID: 29386552
- PMCID: PMC5792543
- DOI: 10.1038/s41598-018-20390-5
We aimed to elucidate the effects of iguratimod, a widely used anti-rheumatic drug with no severe side effects, on chronic experimental autoimmune encephalomyelitis (EAE), an animal model of multiple sclerosis (MS). Iguratimod was orally administered to mice immunised with myelin oligodendrocyte glycoprotein peptide 35-55. Preventive administration of iguratimod from the time of immunisation was found to markedly reduce the clinical severity of acute and chronic EAE. Pathologically, iguratimod treatment significantly reduced demyelination and infiltration of CD3 + T, F4/80 + , and CD169 + cells into the spinal cord, and suppressed macrophage/microglia activation in the parenchyma at the acute and chronic stages compared with vehicle treatment. Therapeutic administration of iguratimod after the onset of clinical symptoms significantly ameliorated the clinical severity of chronic EAE and reduced demyelination, T helper (Th)1/Th17 cell infiltration, macrophage/microglia activation, and nuclear factor (NF)-κB p65 and cyclooxygenase-2 expression in the spinal cord. In vitro, iguratimod treatment inhibited nuclear translocation of NF-κB p65 and down-regulated pro-inflammatory responses in macrophages and microglia. Our results suggest that iguratimod ameliorates acute and chronic EAE by suppressing inflammatory cell infiltration and immune cell activation, partly through inhibition of NF-κB p65, supporting the therapeutic potential of this drug for not only acute, but also chronic MS.
Publication types
- Research Support, Non-U.S. Gov't
- Antirheumatic Agents / administration & dosage
- Antirheumatic Agents / pharmacology
- Antirheumatic Agents / therapeutic use*
- Chromones / administration & dosage
- Chromones / pharmacology
- Chromones / therapeutic use*
- Chronic Disease
- Cyclooxygenase 2 / metabolism
- Down-Regulation* / drug effects
- Encephalomyelitis, Autoimmune, Experimental / drug therapy*
- Encephalomyelitis, Autoimmune, Experimental / immunology
- Encephalomyelitis, Autoimmune, Experimental / pathology
- Immunization
- Inflammation / pathology
- Leukocytes / pathology
- Lipopolysaccharides
- Lymphocyte Activation / drug effects
- Macrophage Activation / drug effects
- Macrophages / drug effects
- Macrophages / metabolism*
- Macrophages / pathology
- Mice, Inbred C57BL
- Microglia / drug effects
- Microglia / metabolism*
- Microglia / pathology
- Myelin-Oligodendrocyte Glycoprotein
- NF-kappa B / metabolism*
- Peptide Fragments
- Signal Transduction*
- Spinal Cord / metabolism
- Spinal Cord / pathology
- Spleen / drug effects
- Spleen / pathology
- Sulfonamides / administration & dosage
- Sulfonamides / pharmacology
- Sulfonamides / therapeutic use*
- Th1 Cells / drug effects
- Th1 Cells / immunology
- Th17 Cells / drug effects
- Th17 Cells / immunology
- Up-Regulation / drug effects
- Antirheumatic Agents
- Sulfonamides
- myelin oligodendrocyte glycoprotein (35-55)
- Cyclooxygenase 2
Thank you for visiting nature.com. You are using a browser version with limited support for CSS. To obtain the best experience, we recommend you use a more up to date browser (or turn off compatibility mode in Internet Explorer). In the meantime, to ensure continued support, we are displaying the site without styles and JavaScript.
- View all journals
- My Account Login
- Explore content
- About the journal
- Publish with us
- Sign up for alerts
- Open access
- Published: 02 September 2015
18β-glycyrrhetinic acid suppresses experimental autoimmune encephalomyelitis through inhibition of microglia activation and promotion of remyelination
- Jieru Zhou 1 , 2 na1 ,
- Wei Cai 3 na1 ,
- Min Jin 2 , 4 na1 ,
- Jingwei Xu 4 na1 ,
- Yanan Wang 4 na1 ,
- Yichuan Xiao 4 na1 ,
- Li Hao 5 na1 ,
- Bei Wang 4 na1 ,
- Yanyun Zhang 4 na1 ,
- Jie Han 1 na1 &
- Rui Huang 2 na1
Scientific Reports volume 5 , Article number: 13713 ( 2015 ) Cite this article
3046 Accesses
40 Citations
1 Altmetric
Metrics details
- Autoimmunity
- Neuroimmunology
Microglia are intrinsic immune cells in the central nervous system (CNS). The under controlled microglia activation plays important roles in inflammatory demyelination diseases, such as multiple sclerosis (MS). However, the means to modulate microglia activation as a therapeutic modality and the underlying mechanisms remain elusive. Here we show that administration of 18β-glycyrrhetinic acid (GRA), by using both preventive and therapeutic treatment protocols, significantly suppresses disease severity of experimental autoimmune encephalomyelitis (EAE) in C57BL/6 mice. The treatment effect of GRA on EAE is attributed to its regulatory effect on microglia. GRA-modulated microglia significantly decreased pro-inflammatory profile in the CNS through suppression of MAPK signal pathway. The ameliorated CNS pro-inflammatory profile prevented the recruitment of encephalitogenic T cells into the CNS, which alleviated inflammation-induced demyelination. In addition, GRA treatment promoted remyelination in the CNS of EAE mice. The induced remyelination can be mediated by the overcome of inflammation-induced blockade of brain-derived neurotrophic factor expression in microglia, as well as enhancing oligodendrocyte precursor cell proliferation. Collectively, our results demonstrate that GRA-modulated microglia suppresses EAE through inhibiting microglia activation-mediated CNS inflammation and promoting neuroprotective effect of microglia, which represents a potential therapeutic strategy for MS and maybe other neuroinflammatory diseases associated with microglia activation.
Similar content being viewed by others
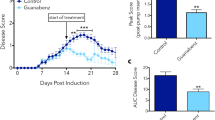
Guanabenz modulates microglia and macrophages during demyelination
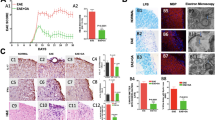
Immunomodulatory therapy with glatiramer acetate reduces endoplasmic reticulum stress and mitochondrial dysfunction in experimental autoimmune encephalomyelitis

Inhibition of the NLRP3-inflammasome prevents cognitive deficits in experimental autoimmune encephalomyelitis mice via the alteration of astrocyte phenotype
Introduction.
Microglia are central nervous system (CNS)-specific macrophages that viewed as the major immunocompetent element in the CNS in charge of sensing any brain-damaging event 1 , 2 . Increasing studies suggest that activation of microglia is a hallmark of inflammatory demyelinating diseases such as multiple sclerosis (MS) and the animal model, experimental autoimmune encephalomyelitis (EAE) 3 , 4 . In MS and EAE, microglia exhibit uncontrolled activation, produce pro-inflammatory mediators, which recruit encephalitogenic T cells into the CNS and play a leading role in oligodendrocyte death and demyelination 5 , 6 , 7 . However, when microglia activation is properly modulated, they can promote CNS remyelination through increased neurotrophic factor production 8 , 9 , 10 , which is in accordance with our recent results 11 . Therefore, the development of new therapeutic approaches designed to modulate activation of microglia, while preserving their neuroprotective effects, would suppress EAE pathogenesis and be great beneficial for MS therapy.
To this end, we employed such an approach to identify novel therapeutic compounds for EAE and to characterize the underlying regulatory mechanisms. We recently find that 18β-glycyrrhetinic acid (GRA), a chemically defined compound, shows a potent inhibitory effect on the inflammatory activation of liver-resident macrophages, Kupffer cells 12 . In addition, GRA exhibits neuroprotective effects 13 , which prompted us to examine whether GRA has potential regulatory effects on modulation of CNS-resident macrophages, microglia in EAE. Our data indicate that GRA effectively suppresses EAE disease severity and the treatment effect is attributed to GRA-modulated microglia, which reduce the recruitment of encephalitogenic T cells in the CNS, as well as promote oligodendrocyte precursor cell (OPC)-mediated CNS remyelination.
GRA reduces CNS inflammation and myelin damage in EAE
To explore the efficacy of GRA, a natural pentacyclic triterpene ( Fig. 1a ) on actively induced EAE, GRA or vehicle control was administered i.p. daily in MOG 35–55 -immunized mice beginning on two different days: at day 7 as a preventive treatment protocol, when no clinical symptoms were observed; and at day 11 as a therapeutic treatment protocol. As illustrated in Fig. 1b and Fig. S1a when administered daily from day 7-post immunization onwards, GRA with the optimal dose 75 mg/kg showed a significant inhibitory effect on the severity of EAE as compared with the vehicle control. The clinical effects became overt at the time of disease onset (day 15) and persisted over the course of EAE. Similar efficacy was observed following the therapeutic treatment protocol ( Fig. 1c ). The effects of the treatment starting at day 15-post immunization was not as prominent as that of day 11-post immunization although mice attained clinical scores close to the maximum severity at day 15-post immunization ( Fig. S1b ).
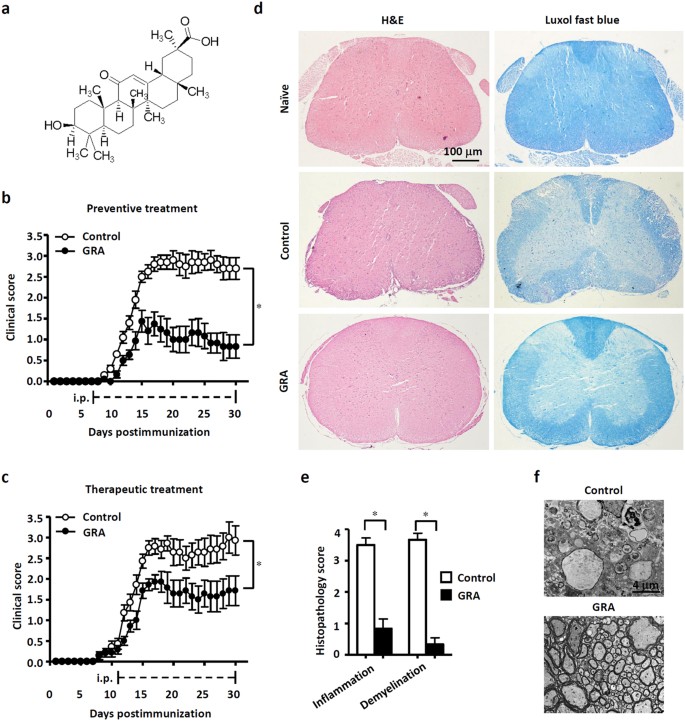
GRA reduces CNS inflammation and myelin damage in EAE.
( a ) Chemical structure of GRA. ( b , c ) Clinical scores of EAE mice that were i.p. injected daily with GRA (75 mg/kg) or vehicle control from day 7 (n = 10, preventive treatment) or day 11 (n = 7, therapeutic treatment)-post immunization onwards. ( d ) Transverse sections of spinal cords from control EAE mice and GRA-treated mice were stained with H&E or Luxol fast blue (×40). ( e ) Histopathology score of CNS inflammation and demyelination was quantified using H&E and Loxol fast blue staining on day 20 post immunization. ( f ) TEM images showed demyelinated axons in the spinal cords of control EAE and GRA-treated mice on day 25. Data are representative of three independent experiments. * P < 0.05.
To further differentiate pathological changes in EAE upon GRA treatment, we performed histologic analyses to examine CNS inflammatory infiltration and demyelination using the preventive treatment protocol. As shown in Fig. 1d,e , spinal cords from GRA-treated mice contained much lower incidences of both CNS inflammatory cell infiltration and demyelinated regions in the white matter compared with control EAE mice. In addition, transmission electron microscopy (TEM) showed that lower EAE scores did indeed reflect decreased myelin damage in GRA-treated mice ( Fig. 1f ). Taken together, these results indicate that GRA effectively reduces EAE severity by suppressing CNS inflammation and demyelination.
GRA prevents the recruitment of encephalitogenic T cells into the CNS in EAE
To investigate the mechanisms by which in vivo administration of GRA attenuated disease severity of EAE, day 15 splenocytes were isolated from GRA-treated and control EAE mice and characterized for ex vivo T cell reactivity and cytokine profile in response to MOG challenge. The results revealed that the proliferation of MOG-reactive T cells derived from GRA-treated mice was similar to that of controls ( Fig. 2a ). In addition, there was no significant difference in the amounts of cytokines (IFN-γ, IL-4, IL-10 and IL-17) released by T cells obtained upon MOG stimulation of GRA-treated or control EAE mice ( Fig. 2b ). Moreover, GRA treatment did not alter the proportion and quantity of T helper (Th) 1 cells, Th17 and regulatory T cells (Treg) in EAE mice ( Fig. 2c ). Recent studies report that granulocyte-macrophage colony-stimulating factor (GM-CSF) in the encephalitogenicity of T cells is involved in EAE development 14 , 15 . As expected, GRA treatment did not alter the proportion and quantity of GM-CSF + T cells in accordance to IFN-γ + and IL-17 + T cells in EAE mice ( Fig. 2c ).
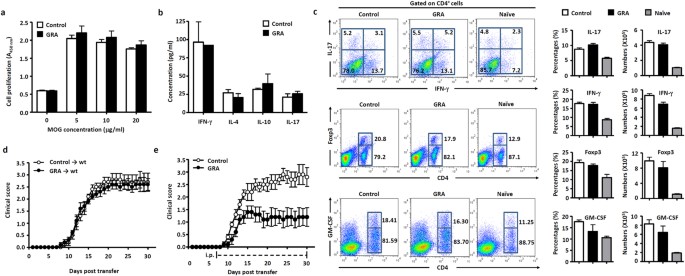
GRA prevents the recruitment of encephalitogenic T cells into the CNS in EAE.
( a – c ) Splenocytes from naïve, control EAE and GRA-treated mice at day 15 following the therapeutic treatment protocol were stimulated with MOG ex vivo and proliferation of MOG-reactive T cells, cytokine profile and proportion of Th1, Th17 and Treg were analyzed. Representative dot plots were shown on the left, percentages and absolute numbers of cells were shown on the right (n = 6). ( d ) DLN cells and splenocytes from control EAE (n = 5) or GRA-treated mice (n = 5) at day 10 were transferred into sublethally irradiated mice. Mice were monitored and scored daily. ( e ) DLN cells and splenocytes from day 10 EAE mice were cultured with MOG and then transferred into sublethally irradiated mice. Recipients were treated with GRA (n = 5) or vehicle control (n = 5) from day 7 post transfer onwards. Mice were monitored and scored daily. Data are representative of three independent experiments.
We next examined the ability of encephalitogenic T cells from GRA-treated and control MOG-immunized mice to confer EAE through adoptive transfer. Cells of draining lymph nodes (DLN) and splenocytes from GRA-treated or control EAE mice were adoptively transferred by i.v. injection into sublethally irradiated mice. As shown in Fig. 2d , mice from both groups developed typical EAE disease, with no significant difference in severity. These results indicate that the ameliorated EAE pathology conferred by GRA is not caused by an impaired encephalitogenic T cell response in the periphery.
The absence of an effect of GRA on the encephalitogenic T cell response prompted us to examine whether GRA affects the migration of encephalitogenic T cells into CNS. To examine this issue, activated MOG-specific T cells were obtained by coculture of MOG peptide with DLN cells and splenocytes from EAE mice. Passive EAE was established by transferring the activated MOG-specific T cells through i.v. injection into sublethally irradiated mice that were then treated i.p. with GRA or vehicle control. The result showed that GRA significantly inhibited the disease severity of passive EAE ( Fig. 2e ). This implies that GRA prevents the recruitment of encephalitogenic T cells into the CNS in EAE.
GRA inhibits inflammatory infiltration through suppressed chemokine expression in the CNS of EAE mice
We performed immunohistochemistry to detect CD4 + T cell infiltration in the CNS. As shown in Fig. 3A , in control EAE mice, there were significantly more CD4 + T cells in the spinal cords than that in GRA-treated EAE mice. In addition, the infiltration of encephalitogenic T cells (CD4 + CD45 + and CD8 + CD45 + T cells) in the CNS of GRA-treated mice were significantly decreased as compared with that in control EAE mice ( Fig. 3b ). Besides GRA treatment decreased the proportion and quantity of activated effector T cells in CNS ( Fig. 3c ). The above results indicated the impaired infiltration of encephalitogenic T cells into the CNS as a prominent feature under GRA treatment in EAE mice. The mechanisms underlying the CNS recruitment of encephalitogenic T cells depend on the release of chemotactic mediators and the expression of cell adhersion molecules on endothelial cells in the CNS. However, in this study, GRA showed no significant effect on the expression of adhersion molecules, such as ICAM-1 and VCAM-1 in the CNS of EAE mice 16 ( Fig. 3d ).
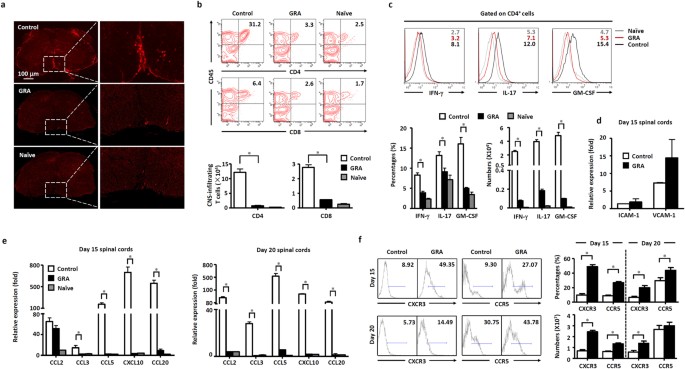
GRA inhibits chemokine expression and proinflammtory infiltration of T cells in the CNS of EAE mice.
( a ) Representative images of CD4 T cell infiltration in day 15 spinal cords of naïve, control and GRA-treated EAE mice following the therapeutic treatment protocol. ( b ) CNS-infiltrating T cells isolated from spinal cords from naïve, control EAE and GRA-treated mice at day 15 were analyzed. Representative dot plots were shown on the upper panel percentages and absolute numbers of cells are shown on the bottom panel (n = 6). ( c ) Spinal cords were isolated from naïve, control EAE and GRA-treated mice at day 15, surface marker and intracellular expression of cytokines were analyzed. Representative dot plots were shown on the upper panel, percentages and absolute numbers of cells were shown on the bottom panel (n = 6). ( d ) Quantification of mRNA abundance for ICAM-1 and VCAM-1 in the CNS from day 15 control and GRA-treated EAE mice. ( e ) Quantification of mRNA abundance for chemokines in the CNS from day 15 and 20 control and GRA-treated EAE mice. ( f ) The proportion of CXCR3 + CD4 + and CCR5 + CD4 + T cells in DLN cells from day 15 and 20 control and GRA-treated EAE mice. Representative dot plots were shown on the left, percentages and absolute numbers of cells were shown on the right (n = 6). Data are representative of three independent experiments. * P < 0.05.
Chemokines, including CCL2, CCL3, CCL5, CXCL10 and CCL20 play important roles in migration of immune cells into the CNS during EAE pathogenesis 17 . We found that the expression of CCL2, CCL3, CCL5, CXCL10 and CCL20 in the CNS reduced significantly in GRA-treated mice compared with control group among which the most conspicuous being CCL5 and CXCL10 ( Fig. 3e ). Accordingly, the proportion and absolute number of CXCR3 + /CCR5 + CD4 + encephalitogenic T cells were significantly higher in the DLN of day 15 and day 20 GRA-treated mice than in control EAE mice ( Fig. 3f ). These results indicate the migration of encephalitogenic T cells into the CNS was inhibited by GRA treatment through suppressed CNS chemokine expression.
GRA inhibits microglia activation through suppression of MAPK signal
In EAE, activated microglia produce increased neurotoxic pro-inflammatory cytokines and chemokines that promote inflammatory infiltration in the CNS 18 , 19 . As GRA-treated mice present a normal immune response in the periphery, while decreased inflammatory profiles in the CNS, we hypothesized that the resistance of these mice to EAE pathology might be due to impaired pro-inflammatory activities in CNS-resident cells such as microglia or in infiltrating macrophage.
To characterize the cellular infiltration in the CNS of EAE mice and to determine which cell populations were altered by GRA treatment, we used chimeric mice that reconstituted with GFP bone marrow (Fig. S2) to distinguish between infiltrating macrophages (CD11b + GFP + ) and CNS-resident microglia (CD11b + GFP − ). To evaluate whether GRA treatment resulted in decreased recruitment of macrophages of peripheral origin, we detected the proportion of CNS-derived GFP + CD11b + cells. The results showed that the proportion of CNS-derived GFP + CD11b + cells has no difference between control and GRA-treated mice, but the absolute numbers of CNS-infiltrating GFP + CD11b + peripheral macrophages are actually lower in GRA-treated mice as compared to control EAE mice. GRA treatment alleviated the autoimmune inflammation in the CNS, leading to dramatically decreased infiltration total mononuclear into CNS. However, GRA treatment did not alter the proportion and pattern of infiltrated mononuclear cell subpopulation (Fig. S3a). These data suggest that the therapeutic effect of GRA may related with the activated microglia but not infiltrating macrophages.
We detected microglial activation depend on the acquisition of markers CD45 and MHC class ΙΙ (MHC ΙΙ) 20 . After EAE induction, control chimeras had a substantially increased frequency of activated microglia (CD45 + MHC ΙΙ + GFP − ) and a decreased frequency of resting microglia (CD45 − MHC ΙΙ − GFP − ), whereas the GRA treatment chimeras contained predominantly resting microglia ( Fig. 4a ), suggesting impaired microglia activation status in GRA-treated mice. Besides, microglia isolated from GRA treatment chimera mice, also had impaired expression of pro-inflammatory cytokines compared with the control ( Fig. 4b ). The active microglia presented long cisternae of granular endoplasmic reticulum and more irregular cell body shapes and exhibited phagocytosis of destroyed myelin and cellular debris. GRA treatment suppressed phagocytosis by microglia, as characterized by fewer fragments in the cytoplasm, suggesting impaired microglia activation ( Fig. 4c ). In control EAE mice, activated microglia with more irregular cell body shapes gather together in demyelinated regions, showing enhanced phagocytic ability in engulfment of damaged cells and cellular parts. By contrast, microglia were dispersed in GRA-treated mice, showing suppressed activation status. To confirm the inhibitory effect of GRA on microglia activation, primary cultured microglia with approximately 95% purity were prepared. Resting microglia presented a dendritic-like morphology with highly ramified processes ( Fig. 4d ). While activated with IFN-γ, microglia became amoeboid in shape. However, GRA addition reversed IFN-γ-induced microglia activation and these cells exhibited ramified cytoplasmic processes of various degrees in microglia.
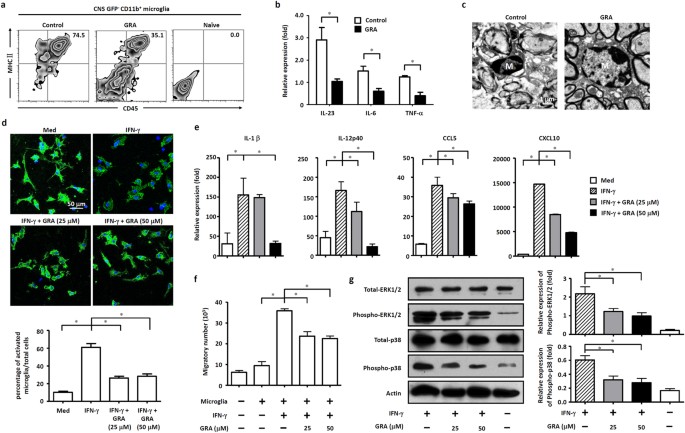
GRA inhibits microglia activation through suppression of MAPK signal pathway.
( a ) Flow cytometry analysis of activation markers (CD45 and MHC ΙΙ) on gated GFP − CD11b + microglia isolated from the CNS of naïve, control and GRA treatment GFP-chimeric mice assessed on 15 day post immunization. Data are representative of four mice per group. ( b ) qPCR analysis of the indicated genes in FACS-sorted GPF − CD11b + microglia isolated from naïve, control and GRA treatment GFP-chimeric EAE mice on 15 day post immunization. ( c ) TEM analyses of microglia status in spinal cords of day 15 control and GRA-treated EAE mice. ( d ) Representative images of primary microglia cultured with medium alone or treated with IFN-γ (100 ng/ml) or IFN-γ (100 ng/ml) plus GRA at the indicated concentrations and the percentage of activated microglia with amoeboid shape normalized to total microglia (CD11b + ). CD11b, green; DAPI, blue. * P < 0.05. ( e ) Primary microglia were cultured with medium alone or treated with IFN-γ (100 ng/ml) or IFN-γ (100 ng/ml) plus GRA at the indicated concentrations. Quantification of mRNA abundance for IL-1β, IL-12p40, CCL5 and CXCL10. ( f ) Culture supernatants were collected and cocultured with DLN cells isolated from day 15 EAE mice. The chemotactic ability was analyzed by counting the amount of migratory cells in the lower chamber. ( g ) Protein extracts from cultured microglia for 48 h were analyzed by western blot for expression of phosphorylated ERK1/2 and p38. Quantification results were shown on the right. Data are representative of three independent experiments. * P < 0.05.
The inhibitory effect of GRA on IFN-γ-induced activation of microglia encouraged us to investigate the underlying mechanisms. We investigated the expression of several activation-associated, pro-inflammatory genes in primary microglia subjected to various treatments. Results revealed that the mRNA abundance for IL-1β, IL-12, CCL5 and CXCL10 was significantly up-regulated in IFN-γ-treated microglia. However, the up-regulation was inhibited by GRA addition to microglia cultures in a dose-dependent manner ( Fig. 4e ). In line with these findings, the migration of encephalitogenic T cells to the supernatants from IFN-γ induced GRA-treated primary microglia cultures were markedly inhibited as compared with that from IFN-γ-induced primary microglia cultures ( Fig. 4f ). However, GRA didn’t affect the activation of macrophage, or marginal effect (Fig. S3b,c). These results indicate that GRA suppresses CNS pro-inflammatory profile through inhibition of microglia activation during EAE pathogenesis.
The MAPK signaling transduction pathway plays an important role in IFN-γ-induced expression of pro-inflammatory genes in activated microglia 21 , 22 . Western blot analyses showed that IFN-γ induced marked elevation of phosphorylation of ERK1/2 and p38 in microglia. However, the elevation can be blocked by GRA in a dose-dependent manner ( Fig. 4g ). These results demonstrate that GRA decreases microglia production of pro-inflammatory cytokines and chemokines and the inhibitory effect can be mediated by suppression of IFN-γ-induced activation via the MAPK signal pathway.
GRA promotes remyelination in EAE
The amelioration of EAE pathology results from two major aspects of function, the suppression of CNS inflammation and the promotion of remyelination 23 , 24 . We then examined whether GRA treatment played roles in CNS remyelination. Previous studies revealed that the expression of myelin genes, such as myelin basic protein (MBP) and proteolipid protein (PLP), increased during remyelination and this early alteration in gene expression is believed to reflect an important step leading to the remyelination process 25 , 26 . As illustrated in Fig. 5a , the expression of MBP was almost at the same level at day 10 in the CNS of GRA-treated and control EAE mice. Although the expression of MBP decreased sharply both in GRA-treated and control group at day 15, MBP expression was significantly higher in GRA-treated mice than in control EAE mice. On day 20, the expression of MBP up-regulated in both groups, but could not return to the level of day 10 in control EAE mice, which indicated permanent damage in the CNS. By contrast, GRA treatment led to significant recovery of MBP expression at day 20. Likewise, similar trends in PLP expression were observed in control and GRA-treated mice ( Fig. 5b ). In addition, immunohistochemical analyses revealed significant up-regulated expression of MBP in spinal cords of day 35 GRA-treated mice ( Fig. 5c ). These results suggest that GRA may promote the remyelination process in EAE mice.
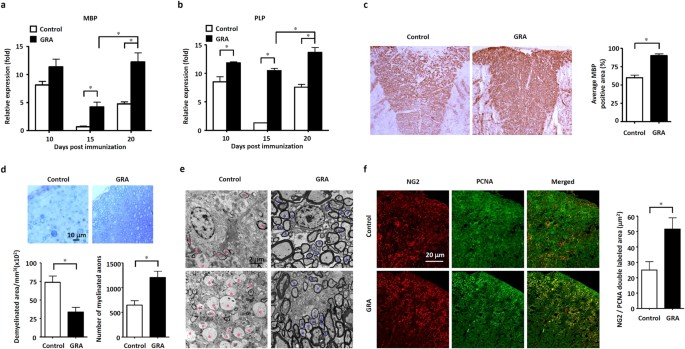
GRA promotes remyelination in EAE.
( a , b ) Quantification of mRNA abundance for MBP and PLP in the CNS from control and GRA-treated EAE mice at day 10, 15 and 20 following therapeutic treatment protocol (n = 6). ( c ) Representative images of MBP immunostaining in spinal cords from control EAE and GRA-treated mice at day 35 and quantification of MBP positively stained areas in spinal cords of GRA-treated and control group. ( d ) Toluidine blue staining of myelinated axons in day 30 spinal cords from control EAE and GRA-treated mice and quantification of demyelinated areas and the number of myelinated axons in GRA-treated and control EAE mice using toluidine staining. ( e ) TEM showing demyelinated axons (red asterisks) in control spinal cords and different remyelination phases with newly formed myelinated axons (blue asterisks) in spinal cords from GRA-treated day 30 EAE mice. ( f ) Spinal cords from day 30 GRA-treated and control EAE mice were stained with NG2 and PCNA for analyses of the proliferation of OPCs. Data are representative of three independent experiments. * P < 0.05.
We then used toluidine blue staining and TEM to determine myelin status in EAE mice. On day 30 in the CNS of GRA-treated mice, large amounts of thinly myelinated axons were observed around demyelinated regions ( Fig. 5d ). Different remyelination phases were observed under TEM analyses. In some visual fields, oligodendrocytes (with rounded or oval profile and few visible processes) were seen in close contact with newly-formed thinly myelinated axons ( Fig. 5e ). The presence of thinly myelinated axons in demyelinated regions provides strong evidence of axon remyelination under GRA treatment 27 .
Remyelination occurs when there are sources of oligodendrocytes, among which most come from OPCs and the crucial first step in OPC-mediated remyelination involves OPC proliferation 23 , 28 . We studied the effect of GRA on the status of OPCs, which were identified by their early phenotypic marker NG2. As shown in Fig. 5F , the number of NG2 + OPCs in the white matter of the spinal cords was markedly increased in day 30 GRA-treated mice compared with that of control EAE mice. Meanwhile, OPC proliferation was evaluated by colocalization of NG2 and PCNA. Immunohistochemical analyses revealed that GRA significantly increased the number of NG2 + PCNA + OPCs in the white matter compared to control EAE mice. These results indicate that GRA induced remyelination in the CNS can be mediated by promotion of OPC proliferation.
GRA-modulated microglia promote brain-derived neurotrophic factor (BDNF) expression
The neurotrophic factor BDNF plays a key role in neuronal and axonal survival, which is partly mediated by promoting OPC-mediated remyelination 29 . Deficiency of BDNF can lead to reduced NG2 + OPCs and decreased expression of MBP and PLP in the CNS 30 . We found similar trends of BDNF expression as MBP and PLP in the CNS of GRA-treated mice ( Fig. 6a ). The ELISA results showed similar trends of BDNF expression ( Fig. 6b ). As microglia are one of the major sources of BDNF in the CNS, we analyzed BDNF expression in primary microglia subjected to various treatments. Results showed that IFN-γ significantly decreased the expression of BDNF, whereas GRA blocked the down-regulation caused by IFN-γ ( Fig. 6c ). However, GRA failed to rescue BDNF down-regulation caused by IFN-γ in astrocytes ( Fig. 6d ), which are also the source of BDNF in the CNS. These results collectively indicate that GRA-induced promotion of OPC proliferation can be mediated by increased BDNF in the CNS.
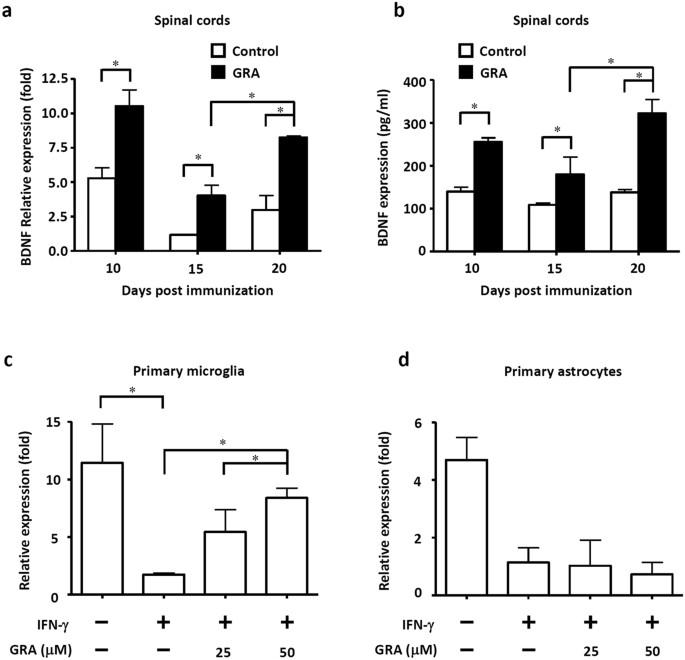
GRA-modulated microglia but not astrocyte exhibit promoted BDNF expression.
( a ) Quantification of mRNA abundance for BDNF in the CNS from control and GRA-treated EAE mice at day 10, 15 and 20 following therapeutic treatment protocol (n = 6). ( b ) Levels of serum BDNF in the CNS from control and GRA-treated EAE mice by ELISA at day 10, 15 and 20 (n = 6). ( c ) Primary microglia were cultured in medium alone or treated with IFN-γ (100 ng/ml) or IFN-γ (100 ng/ml) plus GRA at the indicated concentrations and mRNA abundance for BDNF was quantified. ( d ) Primary astrocytes were cultured in medium alone or treated with IFN-γ (100 ng/ml) or IFN-γ (100 ng/ml) plus GRA at the indicated concentrations and mRNA abundance for BDNF was quantified by real-time PCR. Data are representative of three independent experiments. * P < 0.05.
In MS and EAE, microglia activate rapidly, produce large amounts of pro-inflammatory cytokines and chemokines, which mobilize more immune cells to infiltrate into the CNS and ultimately result in oligodendrocyte death and demyelination 31 . Because microglia serve as the major immunocompetent element in the CNS and the first cells in the CNS to respond to neuronal damage, modulation of microglia function can be a therapeutic strategy in coping CNS inflammation and inflammation-induced demyelination. Here we demonstrate that GRA possesses unique therapeutic effects in EAE, which are mostly attributed to the modulatory effect of GRA on microglia, accompanied by significantly reduced CNS inflammation and myelin damage, as well as promotion of CNS remyelination in EAE mice.
Efficient activation of encephalitogenic T cells is an obligatory requirement for induction of CNS inflammation and pathology in EAE. While in this study, T cells derived from GRA-treated mice did not show any impaired activity as indicated by in vitro recall responses and adoptive transfer model, which further confirmed that GRA did not directly affect peripheral lymphocyte response 12 . However, adoptively transferred EAE mice exhibited suppressed clinical symptoms after treated with GRA, indicating that the alleviated EAE pathology in GRA-treated mice can be due to the prevented recruitment of encephalitogenic T cells into the CNS. Indeed, pro-inflammatory chemokines in the CNS were down-regulated and the infiltration of inflammatory cells were reduced accordingly in GRA-treated mice. As the CNS-resident cells, such as microglia, are major producers of these chemokines in EAE 32 , we assumed that reduced chemokine production in the CNS by GRA may be mediated by modulation of microglia activation. This is proved by observation of the inhibited microglia activation and impaired expression of pro-inflammatory cytokine in the CNS and in vitro cultures after GRA treatment. However, as another group of important cells during EAE, the activation of macrophage wasn’t affected by GRA. GRA can affect cell functions by targeting gap junction channels 33 , 34 , toll-kike receptor 35 , cytochrome P450 2E1(CYP2E1) 36 and so on. It has be regarded that these signalings such as gap junction channels paly critical roles in both microglia macrophages 37 . To explain the different effects of GRA on microglia and macrophages, further investigation is needed. Taken together, our data demonstrated that GRA showed potent ability in modulating microglia activation, leading to suppressed pro-inflammatory profile in the CNS of EAE mice. This alteration reduced the recruitment of encephalitogenic T cells into the CNS and alleviated inflammation-induced demyelination in the CNS. Besides microglia were active in the induction phase of EAE and the therapeutic effects of GRA is because of the inhibition of the microglia activation but not the encephalitogenic T cells. It may explain why day 15-post immunization when the mice developed severe clinical symptoms was not an optimal therapeutic time point.
The expression of myelin genes such as MBP and PLP in the CNS are considered to reflect the dynamic processes of demyelination and remyelination in EAE pathogenesis 25 , 26 . Our results demonstrated that GRA promoted MBP and PLP expression in the CNS during EAE process, together with the observation of the presence of thinly myelinated axons in demyelinated areas, indicating promoted remyelination in the CNS of EAE mice. Because the major source for remyelination in EAE depends on OPCs 23 , 28 , it is reasonable to assume that the promoted remyelination in GRA-treated mice results from the remyelinatory ability of OPCs 38 . Our data indicated that GRA treatment augmented the proliferation of OPCs in the white matter of the CNS. Moreover, the increased colocalization of NG2 and PCNA in the CNS provided strong evidence that GRA treatment had the ability to promote axon remyelination in the CNS in EAE mice.
Increasing studies as well as our previous work, had demonstrated that, properly modulated-microglia had the potential to promote OPC-mediated functional recovery in CNS inflammatory diseases 8 , 9 . BDNF is one of the major neurotrophic factors released by microglia and was reported to promote OPC proliferation and the differentiation of oligodendrocyte 39 , 40 . In addition, BDNF deficiency impaired the proliferation and differentiation of OPCs, leading to reduced NG2 + OPCs and decreased expression of MBP and PLP in the CNS 30 . In EAE mice, the expression of BDNF decreased along with increased EAE severity. However, in GRA-treated mice, BDNF was significantly up-regulated in the CNS, with the expression trend similar to that of MBP and PLP. In addition, GRA overcame the blockade of BDNF expression caused by IFN-γ in primary microglia in vitro . These results indicated that the remyelination process of GRA-treated mice could be attributed to promoted BDNF expression by GRA-modulated microglia.
In conclusion, we demonstrated that GRA had a unique therapeutic potential for EAE through modulation of microglia activation via the inhibition of MAPK signal pathway. GRA-modulated microglia down-regulated CNS pro-inflammatory profile, which reduced the recruitment of encephalitogenic T cells into the CNS and alleviated inflammation-induced demyelination. Importantly, GRA promoted remyelination through augmented OPC proliferation, which could be attributed to the increased BDNF in the CNS by GRA-modulated microglia. This study hopefully provides a new potential therapeutic approach to MS or maybe other neurological diseases based on modulation of microglia.
Ethics Statement
All animal experiments were performed in accordance with the guidelines from the Biomedical Research Ethics Committee of Shanghai Institutes for Biological Sciences, Chinese Academy of Sciences. The protocol used was reviewed and approved by the Animal Experiment Administration Committee of the Biomedical Research Ethics Committee of Shanghai Institutes for Biological Sciences, Chinese Academy of Sciences. All efforts were made to minimize the suffering of mice during experiments.
Induction and treatment of EAE
Female C57BL/6 mice were purchased from Shanghai Laboratory Animal Center, Chinese Academy of Sciences and were kept under pathogen-free condition in the animal center of the Shanghai Jiao Tong University School of Medicine (Shanghai, China). Mice (6–8 weeks of age) were immunized s.c. with the synthetic peptide (300 μg) MOG 35–55 (MEVGWYRSPFSRVVHLYRNGK) (GL Biochem, Shanghai, China). Immunization was performed by mixing MOG peptide in CFA (Sigma-Aldrich, St. louis, MO, USA) containing 5 mg/ml Mycobacterium tuberculosis H37Ra (Difco Laboratories, Detroit, MI, USA). Pertussis toxin (200 ng; List Biological Laboratories, Campbell, CA, USA) in PBS was administered i.v. on days 0 and 2. For treatment of EAE, GRA (Sigma-Aldrich) or DMSO (Sigma-Aldrich) as vehicle control was administered at 75 mg/kg i.p. daily from day 7 or day 11 post-immunization onwards. For adoptively transferred EAE, splenocytes were harvested from EAE mice on day 10 post-immunization. The cells (5 × 10 6 cells/ml) were cultured with MOG 35–55 peptide (20 μg/ml) for 4 days and were then i.v. transferred into sublethally (500 rad) irradiated mice (2 × 10 7 cells/mouse). The recipients received 200 ng of pertussis toxin by i.v. injection immediately after cell transferation and 2 days later. Recipients were also treated i.p. daily with GRA or vehicle control from day 7 of transfer onwards. In some experiments, mice were immunized s.c. with 300 μg of MOG 35–55 emulsified in CFA and treated with GRA or vehicle control on the day of the first immunization. Ten days after immunization, cells from DLN (subiliac, proper axillary and accessory axillary) 41 and spleens were isolated, pooled and directly transferred into sublethally (500 rad) irradiated mice (2 × 10 7 cells/mouse). The recipients received 200 ng of pertussis toxin by i.v. injection immediately after cell transfer and 2 days later. Mice were examined daily and scored for disease severity using the standard scale: 0, no clinical signs; 1, limp tail; 2, paraparesis (weakness, incomplete paralysis of one or two hind limbs); 3, paraplegia (complete paralysis of two hind limbs); 4, paraplegia with forelimb weakness or paralysis; 5, moribund or death. After the onset of EAE, food and water were provided on the cage floor. To eliminate any diagnostic bias, scores were assigned by researchers blinded to mouse identity. 50 mg/kg BrdU was injected intraperitoneally for 3 d before sacrificed to label dividing cells.
Bone marrow chimeras
GFP-transgenic (C57BL/6-Tg (CAG-EGFP) 10sb/J) mice were purchased from from The Jackson Laboratory. We adoptively transferred lethally irradiated (950 rad) C57BL/6 mice (8 weeks of age) with mononuclear bone marrow cells (1 × 10 7 ) derived from the GFP-transgenic mice. Under these conditions, the radioresistant CNS-resident cells would be retained, whereas the radiosensitive bone marrow and peripheral immune cells would be eliminated and replaced by the GFP + cells. The bone marrow reconstitution does not affect the homeostasis of CNS-resident cells. We immunized the chimeric mice for EAE induction at 8 week after bone marrow reconstitution.
Histopathology
Spinal cords from mice transcardially perfused with 4% (w/v) paraformaldehyde were dissected and postfixed overnight. Paraffin-embedded sections (8 μm) of spinal cords were stained with hematoxylin and eosin (H&E) or Luxol Fast Blue and examined by light microscopy (Nikon, Yurakucho, Tokyo, Japan). Semiquantitative analyses of inflammation and demyelination were performed in a blinded manner as previously described 11 .
Cell proliferation and cytokine assay
Splenocytes isolated from MOG 35–55 -immunized mice were cultured in triplicate in complete DMEM at a density of 5 × 10 5 per well in 96-well plates in the presence or absence of MOG peptide at the indicated concentration and were maintained at 37 °C in 5% CO 2 for 72 h. Cell proliferation was measured with CCK8 reagent (Dojindo Molecular Technologies Inc., Shanghai, China). For cytokine measurements, supernatants were collected from cell cultures at 48 h and diluted for the measurements of IFN-γ, IL-4, IL-10 and IL-17 by ELISA (R&D Systems, Minneapolis, MN, USA) according to the manufacturer’s instructions.
Preparation of CNS mononuclear cells and flow cytometry
For preparation of CNS mononuclear cells, brains and spinal cords from MOG 35–55 -immunized mice were excised and dissociated for 45 min at 37 °C by digestion with collagenase IV (2 mg/ml, Sigmal-Aldrich) and DNase I (100 μg/ml, Sigmal-Aldrich) in DMEM medium. Dispersed cells were passed through a 70 μm nylon mesh and collected by centrifugation. CNS mononuclear cells were isolated through a Percoll density gradient and collected from the interface fraction between 37% and 70% Percoll. After extensive washing, suspensions of cells were stained with FITC-labeled anti-CD4, PE-conjugated anti-CD11b, APC-conjugated anti-CD45, PE-Cy7-conjugated anti-MHC ΙΙ, PE-Cy7-conjugated anti-CD8, PE-conjugated anti-CXCR3, PE-conjugated anti-CCR5 (all from BD Pharmingen, San Diego, CA, USA). Isotype controls were used for determination of negative cells. The stained cells were analyzed on a FACSAria instrument (BD Bioscience, San Diego, CA, USA). For Th1, Th17 and Treg cell analysis, cells were stained with surface marker, permeabilized with the Intracellular Fixation and Permeabilization Buffer Set (eBioscience, San Diego, CA,USA) and then stained with anti IFN-γ, anti-IL17A, GM-CSF and anti-Foxp3 antibodies. All these antibodies were purchased from eBioscience, unless marked otherwise.
Primary microglia culture and T cell coculture assay
Primary microglia cultures were prepared as previously described 11 . Briefly, cerebral cortical cells from newborn C57BL/6 mice were dissociated after a 30-min trypsinization (0.25%) and were plated in 75-cm 2 culture flasks (Corning Inc., Corning, NY, USA) in DMEM supplemented with 10% heat-inactivated fetal calf serum, 100 U/ml penicillin and 100 mg/ml streptomycin. The culture medium was changed after 24 h and then once in every 4 days. Two weeks later, microglia were obtained by mild trypsinization. Purified microglia comprised a cell population in which 95% stained positively with CD11b antibodies. For treatment assay, microglia were incubated with complete DMEM and stimulated with or without 100 ng/ml IFN-γ (R&D) in the presence or absence of GRA (25 μM and 50 μM) at 37 °C in a humidified incubator with 5% CO 2 . For cell migration assay, the isolated primary microglia that seeded in complete DMEM medium were stimulated with or without IFN-γ (100 ng/ml) and treated with different doses of GRA, 24 h later, the microglia culture supernatants were collected and added to the lower chambers of Transwell inserts (Millipore). DLN cells isolated from day 15 EAE mice were seeded into the upper chambers. The migration was carried out for 2 h. After incubation, the nonmigrated cells were removed from the upper surface of the filters and the chemotactic ability was analyzed by counting the amount of migratory cells in the lower chamber.
Quantitative real-time PCR
Total RNA was isolated using TRIzol (Invitrogen, Carlsbad, CA, USA) according to the manufacturer’s instructions and reverse transcribed. mRNA abundance was determined by real-time PCR using SYBR Green master mix (Applied Biosystems, Foster City, CA, USA). Primer sequences are detailed in Table S1 . Data were collected and quantitatively analyzed on an ABI Prism 7900 sequence detection system (Applied Biosystems). The mouse β-actin gene was used as an endogenous control for sample normalization.
Western Blot
Protein extracted from cultured microglia subjected to various treatments were resolved in 12% SDS-polyacrylamide gels and transferred to PVDF membranes. The protein of interest was detected by immunostaining with rabbit anti-phospho-p38, p38, phospho-ERK1/2, ERK1/2 (all from Cell Signaling Technology, Danvers, MA, USA) or rabbit anti-actin (Sigma-Aldrich), followed by incubation with HRP-labeled secondary antibodies and detection with chemiluminescence.
Immunohistochemistry and immunocytochemistry
For immunohistochemistry staining, sections of spinal cords were incubated with rat anti-mouse CD11b (1:50, BD Pharmingen, San Diego, CA, USA), rabbit anti-mouse NG2 Ab (1:100, Chemicon International, USA & Canada), mouse anti-PCNA (1:5000 Abcam, Cambridge, UK,) or mouse anti-MBP (1:1000, Covance Inc., Princeton, New Jersey, USA), which were then labeled with FITC-conjugated goat anti-rat IgG (1:400, Santa Cruz, CA, USA), Cy3-conjugated goat anti-rabbit IgG (1:500, KPL, Kirkegaard & Perry Laboratories, Inc., Gaithersburg, Maryland, USA), Alexa Fluor 488 goat anti-mouse IgG (1:500, Invitrogen) or HRP-conjugated goat anti-mouse IgG (1:1000, KPL) and examined by confocal microscopy (Leica Camera AG, Wetzlar, Germany) or light microscopy (Nikon). For immunocytochemistry staining, microglia were fixed in 4% paraformaldehyde for 20 min, blocked in 1% BSA for 30 min and incubated overnight with rat anti-mouse CD11b (1:50, BD Pharmingen). Subsequently, cells were incubated with FITC-conjugated goat anti-rat secondary antibody (1:400, Santa Cruz). Finally, the cells were counterstained with DAPI and examined under a confocal microscope (Leica).
Toluidine blue staining and EM
Toluidine blue staining and EM analysis were performed as described previously (Xu et al. , 2013). Briefly, mice were anaesthetized and perfused with 0.1 M PBS followed by 0.1 M PBS containing 2.5% glutaraldehyde (pH 7.3). Lumbar spinal cords were sliced into 1mm sections. Thin sections were cut, stained with uranyl acetate and lead citrate and then analyzed under transmission EM (CM-120, Philips, Amsterdam, Netherlands).
Statistical analysis
GraphPad Prism (version 4.0, GraphPad Software, Inc., La Jolla, CA, USA) and SPSS 17.0 software (SPSS Inc. Chicago, IL, USA) was used for statistical analyses. Significant differences were evaluated using an independent-samples t test or Wilcoxon rank test, except that multiple treatment groups were compared within individual experiments by ANOVA test. Kruskal-Wallis is used for EAE curves. Values of P less than 0.05 were considered significant. All values were presented as mean ± SEM.
Additional Information
How to cite this article : Zhou, J. et al. 18β-glycyrrhetinic acid suppresses experimental autoimmune encephalomyelitis through inhibition of microglia activation and promotion of remyelination. Sci. Rep. 5 , 13713; doi: 10.1038/srep13713 (2015).
Nelson, P. T., Soma, L. A. & Lavi, E. Microglia in diseases of the central nervous system. Ann Med 34, 491–500 (2002).
Article CAS Google Scholar
Nimmerjahn, A., Kirchhoff, F. & Helmchen, F. Resting microglial cells are highly dynamic surveillants of brain parenchyma in vivo . Science 308, 1314–8 (2005).
Article ADS CAS Google Scholar
Gonzalez-Scarano, F. & Baltuch, G. Microglia as mediators of inflammatory and degenerative diseases. Annu Rev Neurosci 22, 219–40 (1999).
Yang, G., Parkhurst, C. N., Hayes, S. & Gan, W. B. Peripheral elevation of TNF-alpha leads to early synaptic abnormalities in the mouse somatosensory cortex in experimental autoimmune encephalomyelitis. Proc Natl Acad Sci USA 110, 10306–11 (2013).
Heppner, F. L. et al. Experimental autoimmune encephalomyelitis repressed by microglial paralysis. Nat Med 11, 146–52 (2005).
Kreutzberg, G. W. Microglia: a sensor for pathological events in the CNS. Trends Neurosci 19, 312–8 (1996).
Sorensen, T. L. et al. Expression of specific chemokines and chemokine receptors in the central nervous system of multiple sclerosis patients. J Clin Invest 103, 807–15 (1999).
Butovsky, O. et al. Induction and blockage of oligodendrogenesis by differently activated microglia in an animal model of multiple sclerosis. J Clin Invest 116, 905–15 (2006).
Emmetsberger, J. & Tsirka, S. E. Microglial inhibitory factor (MIF/TKP) mitigates secondary damage following spinal cord injury. Neurobiol Dis 47, 295–309 (2012).
Linker, R. A. et al. Functional role of brain-derived neurotrophic factor in neuroprotective autoimmunity: therapeutic implications in a model of multiple sclerosis. Brain 133, 2248–63 (2010).
Article Google Scholar
Xiao, Y. et al. Genetic ablation of steroid receptor coactivator-3 promotes PPAR-beta-mediated alternative activation of microglia in experimental autoimmune encephalomyelitis. Glia 58, 932–42 (2010).
PubMed PubMed Central Google Scholar
Xiao, Y. et al. 18Beta-glycyrrhetinic acid ameliorates acute Propionibacterium acnes-induced liver injury through inhibition of macrophage inflammatory protein-1alpha. J Biol Chem 285, 1128–37 (2010).
Kao, T. C., Shyu, M. H. & Yen, G. C. Neuroprotective effects of glycyrrhizic acid and 18beta-glycyrrhetinic acid in PC12 cells via modulation of the PI3K/Akt pathway. J Agric Food Chem 57, 754–61 (2009).
Codarri, L. et al. RORgammat drives production of the cytokine GM-CSF in helper T cells, which is essential for the effector phase of autoimmune neuroinflammation. Nat Immunol 12, 560–7 (2011).
El-Behi, M. et al. The encephalitogenicity of T(H)17 cells is dependent on IL-1- and IL-23-induced production of the cytokine GM-CSF. Nat Immunol 12, 568–75 (2011).
Piqueras, L. et al. Activation of PPARbeta/delta inhibits leukocyte recruitment, cell adhesion molecule expression and chemokine release. J Leukoc Biol 86, 115–22 (2009).
Gerard, C. & Rollins, B. J. Chemokines and disease. Nat Immunol 2, 108–15 (2001).
Ambrosini, E. & Aloisi, F. Chemokines and glial cells: a complex network in the central nervous system. Neurochem Res 29, 1017–38 (2004).
Carson, M. J. Microglia as liaisons between the immune and central nervous systems: functional implications for multiple sclerosis. Glia 40, 218–31 (2002).
Ponomarev, E. D., Shriver, L. P., Maresz, K. & Dittel, B. N. Microglial cell activation and proliferation precedes the onset of CNS autoimmunity. J Neurosci Res 81, 374–89 (2005).
Piao, Z. G. et al. Activation of glia and microglial p38 MAPK in medullary dorsal horn contributes to tactile hypersensitivity following trigeminal sensory nerve injury. Pain 121, 219–31 (2006).
Zhou, Y., Ling, E. A. & Dheen, S. T. Dexamethasone suppresses monocyte chemoattractant protein-1 production via mitogen activated protein kinase phosphatase-1 dependent inhibition of Jun N-terminal kinase and p38 mitogen-activated protein kinase in activated rat microglia. J Neurochem 102, 667–78 (2007).
Franklin, R. J. & Ffrench-Constant, C. Remyelination in the CNS: from biology to therapy. Nat Rev Neurosci 9, 839–55 (2008).
Duncan, I. D., Brower, A., Kondo, Y., Curlee, J. F., Jr. & Schultz, R. D. Extensive remyelination of the CNS leads to functional recovery. Proc Natl Acad Sci USA 106, 6832–6 (2009).
Liu, L. et al. Myelin repair is accelerated by inactivating CXCR2 on nonhematopoietic cells. J Neurosci 30, 9074–83 (2010).
Jurevics, H. et al. Alterations in metabolism and gene expression in brain regions during cuprizone-induced demyelination and remyelination. J Neurochem 82, 126–36 (2002).
Mi, S. et al. LINGO-1 antagonist promotes spinal cord remyelination and axonal integrity in MOG-induced experimental autoimmune encephalomyelitis. Nat Med 13, 1228–33 (2007).
Zhang, Y. et al. Notch1 signaling plays a role in regulating precursor differentiation during CNS remyelination. Proc Natl Acad Sci USA 106, 19162–7 (2009).
Girard, C. et al. Grafts of brain-derived neurotrophic factor and neurotrophin 3-transduced primate Schwann cells lead to functional recovery of the demyelinated mouse spinal cord. J Neurosci 25, 7924–33 (2005).
Vondran, M. W., Clinton-Luke, P., Honeywell, J. Z. & Dreyfus, C. F. BDNF+/− mice exhibit deficits in oligodendrocyte lineage cells of the basal forebrain. Glia 58, 848–56 (2010).
Becher, B., Prat, A. & Antel, J. P. Brain-immune connection: immuno-regulatory properties of CNS-resident cells. Glia 29, 293–304 (2000).
Babcock, A. A., Kuziel, W. A., Rivest, S. & Owens, T. Chemokine expression by glial cells directs leukocytes to sites of axonal injury in the CNS. J Neurosci 23, 7922–30 (2003).
Guan, X., Wilson, S., Schlender, K. K. & Ruch, R. J. Gap-junction disassembly and connexin 43 dephosphorylation induced by 18 beta-glycyrrhetinic acid. Mol Carcinog 16, 157–64 (1996).
Yamamoto, Y., Fukuta, H., Nakahira, Y. & Suzuki, H. Blockade by 18beta-glycyrrhetinic acid of intercellular electrical coupling in guinea-pig arterioles. J Physiol 511 (Pt 2), 501–8 (1998).
Gupta, P., Das, P. K. & Ukil, A. Antileishmanial Effect of 18beta-Glycyrrhetinic Acid Is Mediated by Toll-Like Receptor-Dependent Canonical and Noncanonical p38 Activation. Antimicrob Agents Chemother 59, 2531–9 (2015).
Jeong, H. G. et al. Hepatoprotective effects of 18beta-glycyrrhetinic acid on carbon tetrachloride-induced liver injury: inhibition of cytochrome P450 2E1 expression. Pharmacol Res 46, 221–7 (2002).
Eugenin, E. A. et al. The role of gap junction channels during physiologic and pathologic conditions of the human central nervous system. J Neuroimmune Pharmacol 7, 499–518 (2012).
Aharoni, R., Arnon, R. & Eilam, R. Neurogenesis and neuroprotection induced by peripheral immunomodulatory treatment of experimental autoimmune encephalomyelitis. J Neurosci 25, 8217–28 (2005).
Du, Y., Fischer, T. Z., Lee, L. N., Lercher, L. D. & Dreyfus, C. F. Regionally specific effects of BDNF on oligodendrocytes. Dev Neurosci 25, 116–26 (2003).
Du, Y., Fischer, T. Z., Clinton-Luke, P., Lercher, L. D. & Dreyfus, C. F. Distinct effects of p75 in mediating actions of neurotrophins on basal forebrain oligodendrocytes. Mol Cell Neurosci 31, 366–75 (2006).
Stromnes, I. M. & Goverman, J. M. Passive induction of experimental allergic encephalomyelitis. Nat Protoc 1, 1952–60 (2006).
Download references
Acknowledgements
This work was supported by Strategic Priority Research Program of the Chinese Academy of Sciences (XDA01040000), the Ministry of Science and Technology of China (2011CB966200, 2014CB943303), National Natural Science Foundation of China (81130057, 81071748, 81373164, 81190133, 31300708, 81101557, 81470867), the Program of Science and Technology Commission of Shanghai Municipality (12ZR1415900, 13ZR1423200, 14ZR1446300, PKJ-2013-Y10) and Shanghai Leading Academic Discipline Project J50207.
Author information
Zhou Jieru, Cai Wei and Jin Min contributed equally to this work.
Authors and Affiliations
Department of Rheumatology, East Hospital, Tongji University School of Medicine, Shanghai, China
Jieru Zhou & Jie Han
Medical College of Soochow University, Suzhou, Jiangsu, China
Jieru Zhou, Min Jin & Rui Huang
Department of Infectious Diseases, Ruijin Hospital, Shanghai Jiao Tong University School of Medicine, Shanghai, China
Key Laboratory of Stem Cell Biology, Institute of Health Sciences, Shanghai Institutes for Biological Sciences, Chinese Academy of Sciences and Shanghai Jiao Tong University School of Medicine (SJTUSM) and Shanghai Institute of Immunology, Institutes of Medical Sciences, SJTUSM, Shanghai, China
Min Jin, Jingwei Xu, Yanan Wang, Yichuan Xiao, Bei Wang & Yanyun Zhang
Clinical Laboratory, the First Affiliated Hospital of Anhui Medical University, Hefei, China
You can also search for this author in PubMed Google Scholar
Contributions
R.H. and J.H. designed the study and supervised the data analysis. J.Z. and M.J. performed all the experiments and the data analysis. J.X., Y.W., Y.X., L.H. and B.W. contributed to the writing of the manuscript. W.C. and Y.Z. helped with experimental design and data analysis. All authors have reviewed the manuscript.
Ethics declarations
Competing interests.
The authors declare no competing financial interests.
Electronic supplementary material
Supplementary information, rights and permissions.
This work is licensed under a Creative Commons Attribution 4.0 International License. The images or other third party material in this article are included in the article’s Creative Commons license, unless indicated otherwise in the credit line; if the material is not included under the Creative Commons license, users will need to obtain permission from the license holder to reproduce the material. To view a copy of this license, visit http://creativecommons.org/licenses/by/4.0/
Reprints and permissions
About this article
Cite this article.
Zhou, J., Cai, W., Jin, M. et al. 18β-glycyrrhetinic acid suppresses experimental autoimmune encephalomyelitis through inhibition of microglia activation and promotion of remyelination. Sci Rep 5 , 13713 (2015). https://doi.org/10.1038/srep13713
Download citation
Received : 11 March 2015
Accepted : 06 August 2015
Published : 02 September 2015
DOI : https://doi.org/10.1038/srep13713
Share this article
Anyone you share the following link with will be able to read this content:
Sorry, a shareable link is not currently available for this article.
Provided by the Springer Nature SharedIt content-sharing initiative
This article is cited by
18β-glycyrrhetinic acid ameliorates neuroinflammation linked depressive behavior instigated by chronic unpredictable mild stress via triggering bdnf/trkb signaling pathway in rats.
- Girdhari Lal Gupta
- Lalit Sharma
- Manu Sharma
Neurochemical Research (2023)
Neuroprotective Effects of Sinomenine on Experimental Autoimmune Encephalomyelitis via Anti-Inflammatory and Nrf2-Dependent Anti-Oxidative Stress Activity
- Yanhui Yang
NeuroMolecular Medicine (2023)
4-Ethylguaiacol modulates neuroinflammation and Th1/Th17 differentiation to ameliorate disease severity in experimental autoimmune encephalomyelitis
- Wen-Tsan Weng
- Ping-Chang Kuo
- Jui-Hung Yen
Journal of Neuroinflammation (2021)
Neural Stem Cell-Based Regenerative Approaches for the Treatment of Multiple Sclerosis
- Rongbing Yang
- Wenbin Deng
Molecular Neurobiology (2018)
How impaired efficacy happened between Gancao and Yuanhua: Compounds, targets and pathways
- Jianming Guo
- Jin-ao Duan
Scientific Reports (2017)
Quick links
- Explore articles by subject
- Guide to authors
- Editorial policies
Sign up for the Nature Briefing newsletter — what matters in science, free to your inbox daily.

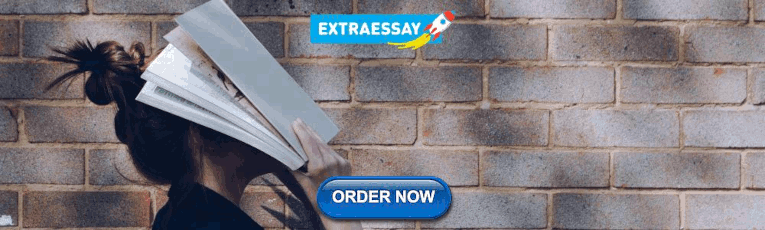
IMAGES
VIDEO
COMMENTS
Suppression of experimental autoimmune encephalomyelitis by interleukin-10 transduced neural stem/progenitor cells. ... Thus, IL-10 is an efficient anti-inflammatory cytokine which potently suppresses EAE and is therefore a suitable candidate molecule to be used in our approach. In addition, the immunomodulatory capacity of IFN-β treatment in ...
A noninflammatory mRNA vaccine for treatment of experimental autoimmune encephalomyelitis. Science 371 , 145-153 (2021). Article PubMed Google Scholar
Multiple sclerosis (MS) is a chronic autoimmune disease of the CNS that is characterized by demyelination, axonal loss, gliosis, and inflammation. The murine model of MS is the experimental autoimmune encephalopathy (EAE) induced by immunization of mice with myelin oligodendrocyte glycoprotein (MOG) 35-55 Ig-like transcript 3 (ILT3) is an ...
A13 suppresses development of experimental autoimmune encephalomyelitis. Mice were immunized with myelin oligodendrocyte glycoprotein peptide (MOG35-55 and injected intraperitoneally (i.p) with ...
Results: A single-cell analysis of brain tissue from mice subjected to experimental autoimmune encephalomyelitis (EAE), a mouse model of CNS inflammation that models aspects of multiple sclerosis (MS), identified microglia with a strong transcriptional signature of senescence including the presence of BCL2-family gene transcripts. Microglia ...
This approach expands a distinct type of antigen-specific effector regulatory T cell that suppresses autoreactivity against targeted autoantigens and promotes bystander suppression of autoreactive T cells against other myelin-specific autoantigens. ... 70 years of merits and culprits in experimental autoimmune encephalomyelitis research. Brain ...
Experimental autoimmune encephalomyelitis (EAE), a widely used animal model that mimics MS, has been extensively used in basic scientific and preclinical studies . ... SF1 suppresses activation of MOG-specific CD4 + T cells in the early onset of EAE. (A) Representative FACS plots of CD4 + naive T, CD4 + Tem, ...
Taken together, our data support the conclusion that sustained ERK1/2 activation in B-cells suppresses immune-mediated demyelination via increasing activation of regulatory B10 cells. Keywords: B10 ... (2016). Experimental autoimmune encephalomyelitis in mice. Methods in Molecular Biology (Clifton, N.J.), 1304, 145-160. [PMC free article ...
Abstract. T helper (Th) cells play crucial roles in inflammation and adaptive immune system. Importantly, Th17 cells, a major pathogenic Th cell subset, are involved in the pathogenesis of multiple sclerosis (MS) and its classical animal modal experimental autoimmune encephalomyelitis (EAE). Previous studies have shown that parthenolide (PTL ...
Certain TLR agonists have been reported to have adjuvant properties in CNS autoimmune inflammatory demyelination. We report in this study that TLR3 stimulation by polyinosinic-polycytidylic acid, a double-stranded RNA analog, suppresses relapsing demyelination in a murine experimental autoimmune encephalomyelitis model.
Background Experimental autoimmune encephalomyelitis (EAE) is a model for inflammatory demyelinating diseases of the central nervous system (CNS), a group of autoimmune diseases characterized by inflammatory infiltration, demyelination, and axonal damage. miR-20a is dysregulated in patients with CNS inflammatory demyelinating diseases; however, the function of miR-20a remains unclear. In this ...
Moreover, NPs carrying ITE and MOG(35-55) expanded the FoxP3(+) Treg compartment and suppressed the development of experimental autoimmune encephalomyelitis, an experimental model of multiple sclerosis. Thus, NPs are potential new tools to induce functional Tregs in autoimmune disorders.
The activation of the NOD-like receptor family pyrin domain-containing protein 3 (NLRP3) inflammasome triggers pyroptosis proinflammatory cell death in experimental autoimmune encephalomyelitis (EAE).
Macrophages play important roles in multiple sclerosis (MS) and experimental autoimmune encephalomyelitis (EAE), and M2 macrophage may have anti-inflammatory effects. In this study, we elucidated the roles of M1 and M2 macrophages in the pathogenesis of EAE and the effects of treatment with M2 macro …
In vivo, treatment with bilirubin effectively suppressed experimental autoimmune encephalomyelitis in SJL/J mice. In contrast, depletion of endogenous bilirubin dramatically exacerbated this disease. In summary, our results identify bilirubin as an important immunomodulator that may protect mammals against autoimmune diseases, thereby ...
Experimental autoimmune encephalomyelitis (EAE) is a widely used animal model for studying human MS, which can help to define the contribution of immune events to the development of MS. Currently, FDA-approved MS therapies act through regulating or inhibiting the peripheral immune response, such as interferon (IFN)-β, dimethyl fumarate ...
Experimental autoimmune encephalomyelitis (EAE), an animal model of MS, is induced by neuroantigen injection and is mediated by CD4 + proinflammatory cells. In acute models of EAE using rats, rabbits, and guinea pigs, pregnancy reduces the incidence of disease and delays the day of onset ( 9 , 10 , 11 ).
Bindarit action on mouse brain and spinal cord in vivo was similarly investigated by qRT-PCR following LPS injection in mice. And to further gauge the potential remedial effects of bindarit on neuroinflammatory disease, its impact on the clinical course of experimental autoimmune encephalomyelitis (EAE) in mice was also explored.
We aimed to elucidate the effects of iguratimod, a widely used anti-rheumatic drug with no severe side effects, on chronic experimental autoimmune encephalomyelitis (EAE), an animal model of multiple sclerosis (MS). Iguratimod was orally administered to mice immunised with myelin oligodendrocyte gly …
Experimental autoimmune encephalomyelitis (EAE) is the animal model of MS for improving understanding and treatment of MS (Rangachari and Kuchroo, 2013). ... IL-33 blockade suppresses the development of experimental autoimmune encephalomyelitis in C57BL/6 mice. J Neuroimmunol, 247 (2012), pp. 25-31.
Li, G., Yamasaki, R., Fang, M. et al. Novel disease-modifying anti-rheumatic drug iguratimod suppresses chronic experimental autoimmune encephalomyelitis by down-regulating activation of ...
Here we show that administration of 18β-glycyrrhetinic acid (GRA), by using both preventive and therapeutic treatment protocols, significantly suppresses disease severity of experimental ...