- To understand the phenomenon Photoelectric effect as a whole.
- To draw kinetic energy of photoelectrons as a function of frequency of incident radiation.
- To determine the Planck's constant from kinetic energy versus frequency graph.
- To plot a graph connecting photocurrent and applied potential.
- To determine the stopping potential from the photocurrent versus applied potential graph.
During his experiments on electromagnetic radiation (to demonstrate light consists of e-m waves), Hertz noticed a spark between the two metallic balls when a high frequency radiation incident on it. This is called photoelectric effect. Photoelectric effect is the emission of electrons when electromagnetic radiations having sufficient frequency incident on certain metal surfaces. We call the emitted electrons as photoelectrons and the current they constitute as photocurrent. The phenomenon was first observed by Heinrich Hertz in 1880 and explained by Albert Einstein in 1905 using Max Planck's quantum theory of light. As the first experiment which demonstrated the quantum theory of energy levels, photoelectric effect experiment is of great historical importance. 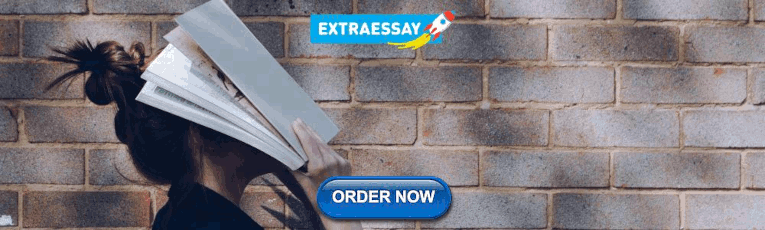 The important observations on Photoelectric effect which demand quantum theory for its explanation are:- The Photoelectric effect is an instantaneous phenomenon. There is no time delay between the incidence of light and emission of photoelectrons.
- The number of photoelectrons emitted is proportional to the intensity of incident light. Also, the energy of emitted photoelectrons is independent of the intensity of incident light.
- The energy of emitted photoelectrons is directly proportional to the frequency of incident light.
The basic experimental set up which explains Photoelectric effect is as given below, .jpg) escape from a particular metal surface and is called work function 'W' for that metal. The work function can be expressed in terms of frequency as, Where h is the Planck's constant and The work function for some metals are listed in the table. According to Einstein the Photoelectric effect should obey the equation, From the above expression,  Graph connecting 'KE max ' and frequency: Maximum kinetic energy of photoelectrons versus frequency of incident radiation graph Now, if we increase the reverse potential, the photocurrent gradually decreases and becomes zero at a particular reverse potential. This minimum applied reverse potential is called stopping potential V 0 . Hence the maximum kinetic energy of photoelectrons can be written as, Graph connecting photocurrent and applied reverse potential :For constant intensity and different frequencies, for constant frequency and different intensities. Cite this Simulator: | Copyright @ 2024 Under the NME ICT initiative of MHRD --> | | - Lecture Demonstration Manual
- Lab Manuals
- Instructional Videos
- PhotoSpheres
You are hereTable of contents. - Experiment 1 - Magnetic Fields of Coils and Faraday's Law
- Experiment 2 - Microwave Optics
- Experiment 3 - Geometrical Optics
- Experiment 4 - Physical Optics
- Experiment 5 - Fluids and Thermodynamics
Experiment 6 - The Photoelectric Effect- Experiment 7 - Radioactivity
- Photodiode with amplifier
- Batteries to operate amplifier and provide reverse voltage
- Digital voltmeter to read reverse voltage
- Source of monochromatic light beams to irradiate photocathode
- Neutral filter to vary light intensity
INTRODUCTION The energy quantization of electromagnetic radiation in general, and of light in particular, is expressed in the famous relation \begin{eqnarray} E &=& hf, \label{eqn_1} \end{eqnarray} where \(E\) is the energy of the radiation, \(f\) is its frequency, and \(h\) is Planck's constant (6.63×10 -34 Js). The notion of light quantization was first introduced by Planck. Its validity is based on solid experimental evidence, most notably the photoelectric effect . The basic physical process underlying this effect is the emission of electrons in metals exposed to light. There are four aspects of photoelectron emission which conflict with the classical view that the instantaneous intensity of electromagnetic radiation is given by the Poynting vector \(\textbf{S}\): \begin{eqnarray} \textbf{S} &=& (\textbf{E}\times\textbf{B})/\mu_0, \label{eqn_2} \end{eqnarray} with \(\textbf{E}\) and \(\textbf{B}\) the electric and magnetic fields of the radiation, respectively, and μ 0 (4π×10 -7 Tm/A) the permeability of free space. Specifically: No photoelectrons are emitted from the metal when the incident light is below a minimum frequency, regardless of its intensity. (The value of the minimum frequency is unique to each metal.) Photoelectrons are emitted from the metal when the incident light is above a threshold frequency. The kinetic energy of the emitted photoelectrons increases with the frequency of the light. The number of emitted photoelectrons increases with the intensity of the incident light. However, the kinetic energy of these electrons is independent of the light intensity. Photoemission is effectively instantaneous. Consider the conduction electrons in a metal to be bound in a well-defined potential. The energy required to release an electron is called the work function \(W_0\) of the metal. In the classical model, a photoelectron could be released if the incident light had sufficient intensity. However, Eq. \eqref{eqn_1} requires that the light exceed a threshold frequency \(f_{\textrm{t}}\) for an electron to be emitted. If \(f > f_{\textrm{t}}\), then a single light quantum (called a photon ) of energy \(E = hf\) is sufficient to liberate an electron, and any residual energy carried by the photon is converted into the kinetic energy of the electron. Thus, from energy conservation, \(E = W_0 + K\), or \begin{eqnarray} K &=& (1/2)mv^2 = E - W_0 = hf - W_0. \label{eqn_3} \end{eqnarray} When the incident light intensity is increased, more photons are available for the release of electrons, and the magnitude of the photoelectric current increases. From Eq. \eqref{eqn_3}, we see that the kinetic energy of the electrons is independent of the light intensity and depends only on the frequency. The photoelectric current in a typical setup is extremely small, and making a precise measurement is difficult. Normally the electrons will reach the anode of the photodiode, and their number can be measured from the (minute) anode current. However, we can apply a reverse voltage to the anode; this reverse voltage repels the electrons and prevents them from reaching the anode. The minimum required voltage is called the stopping potential \(V_{\textrm{s}}\), and the “stopping energy” of each electron is therefore \(eV_{\textrm{s}}\). Thus, \begin{eqnarray} eV_{\textrm{s}} &=& hf - W_0, \label{eqn_4} \end{eqnarray} \begin{eqnarray} V_{\textrm{s}} &=& (h/e)f - W_0/e. \label{eqn_5} \end{eqnarray} Eq. \eqref{eqn_5} shows a linear relationship between the stopping potential \(V_{\textrm{s}}\) and the light frequency \(f\), with slope \(h/e\) and vertical intercept \(-W_0/e\). If the value of the electron charge \(e\) is known, then this equation provides a good method for determining Planck's constant \(h\). In this experiment, we will measure the stopping potential with modern electronics. 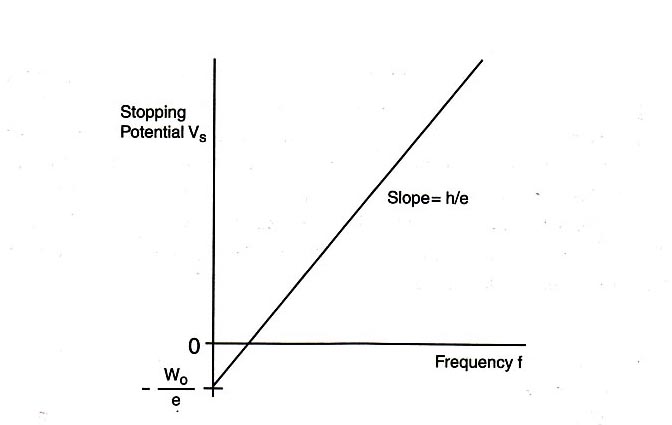 THE PHOTODIODE AND ITS READOUT The central element of the apparatus is the photodiode tube. The diode has a window which allows light to enter, and the cathode is a clean metal surface. To prevent the collision of electrons with air molecules, the diode tube is evacuated. The photodiode and its associated electronics have a small “capacitance” and develop a voltage as they become charged by the emitted electrons. When the voltage across this “capacitor” reaches the stopping potential of the cathode, the voltage difference between the cathode and anode (which is equal to the stopping potential) stabilizes. To measure the stopping potential, we use a very sensitive amplifier which has an input impedance larger than 10 13 ohms. The amplifier enables us to investigate the minuscule number of photoelectrons that are produced. It would take considerable time to discharge the anode at the completion of a measurement by the usual high-leakage resistance of the circuit components, as the input impedance of the amplifier is very high. To speed up this process, a shorting switch is provided; it is labeled “Push to Zero”. The amplifier output will not stay at 0 volts very long after the switch is released. However, the anode output does stabilize once the photoelectrons charge it up. There are two 9-volt batteries already installed in the photodiode housing. To check the batteries, you can use a voltmeter to measure the voltage between the output ground terminal and each battery test terminal. The battery test points are located on the side panel. You should replace the batteries if the voltage is less than 6 volts. THE MONOCHROMATIC LIGHT BEAMS This experiment requires the use of several different monochromatic light beams, which can be obtained from the spectral lines that make up the radiation produced by excited mercury atoms. The light is formed by an electrical discharge in a thin glass tube containing mercury vapor, and harmful ultraviolet components are filtered out by the glass envelope. Mercury light has five narrow spectral lines in the visible region — yellow, green, blue, violet, and ultraviolet — which can be separated spatially by the process of diffraction. For this purpose, we use a high-quality diffraction grating with 6000 lines per centimeter. The desired wavelength is selected with the aid of a collimator, while the intensity can be varied with a set of neutral density filters. A color filter at the entrance of the photodiode is used to minimize room light. The equipment consists of a mercury vapor light housed in a sturdy metal box, which also holds the transformer for the high voltage. The transformer is fed by a 115-volt power source from an ordinary wall outlet. In order to prevent the possibility of getting an electric shock from the high voltage, do not remove the cover from the unit when it is plugged in. To facilitate mounting of the filters, the light box is equipped with rails on the front panel. The optical components include a fixed slit (called a light aperture) which is mounted over the output hole in the front cover of the light box. A lens focuses the aperture on the photodiode window. The diffraction grating is mounted on the same frame that holds the lens, which simplifies the setup somewhat. A “blazed” grating, which has a preferred orientation for maximal light transmission and is not fully symmetric, is used. Turn the grating around to verify that you have the optimal orientation. 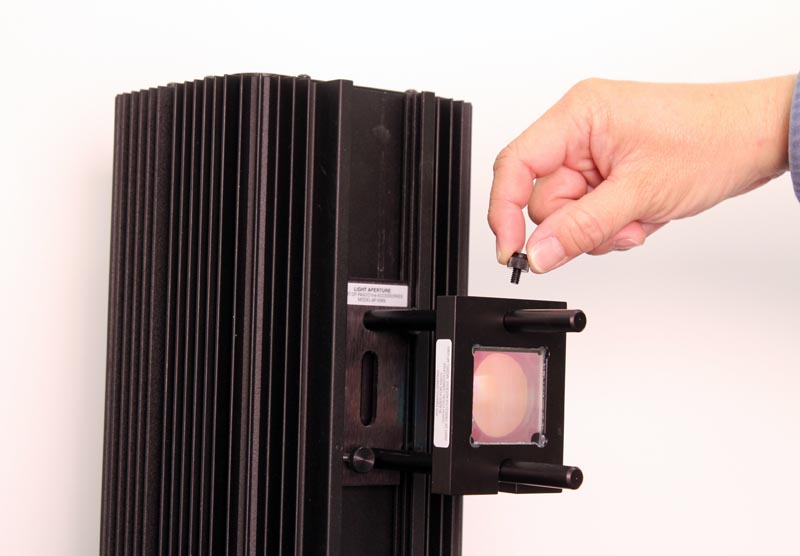 The variable transmission filter consists of computer-generated patterns of dots and lines that vary the intensity of the incident light. The relative transmission percentages are 100%, 80%, 60%, 40%, and 20%. INITIAL SETUP Your apparatus should be set up approximately like the figure above. Turn on the mercury lamp using the switch on the back of the light box. Swing the \(h/e\) apparatus box around on its arm, and you should see at various positions, yellow green, and several blue spectral lines on its front reflective mask. Notice that on one side of the imaginary “front-on” perpendicular line from the mercury lamp, the spectral lines are brighter than the similar lines from the other side. This is because the grating is “blazed”. In you experiments, use the first order spectrum on the side with the brighter lines. 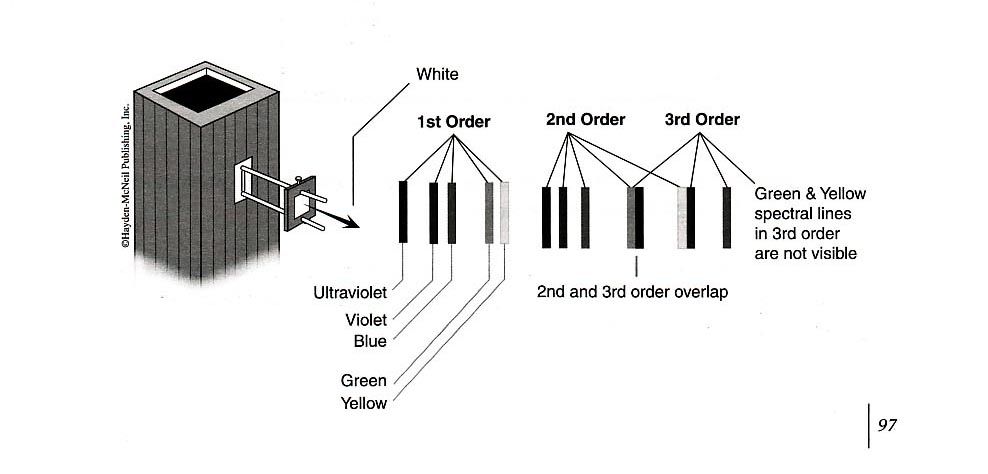 Your apparatus should already be approximately aligned from previous experiments, but make the following alignment checks. Ask you TA for assistance if necessary. Check the alignment of the mercury source and the aperture by looking at the light shining on the back of the grating. If necessary, adjust the back plate of the light-aperture assembly by loosening the two retaining screws and moving the plate to the left or right until the light shines directly on the center of the grating. With the bright colored lines on the front reflective mask, adjust the lens/grating assembly on the mercury lamp light box until the lines are focused as sharply as possible. Roll the round light shield (between the white screen and the photodiode housing) out of the way to view the photodiode window inside the housing. The phototube has a small square window for light to enter. When a spectral line is centered on the front mask, it should also be centered on this window. If not, rotate the housing until the image of the aperture is centered on the window, and fasten the housing. Return the round shield back into position to block stray light. 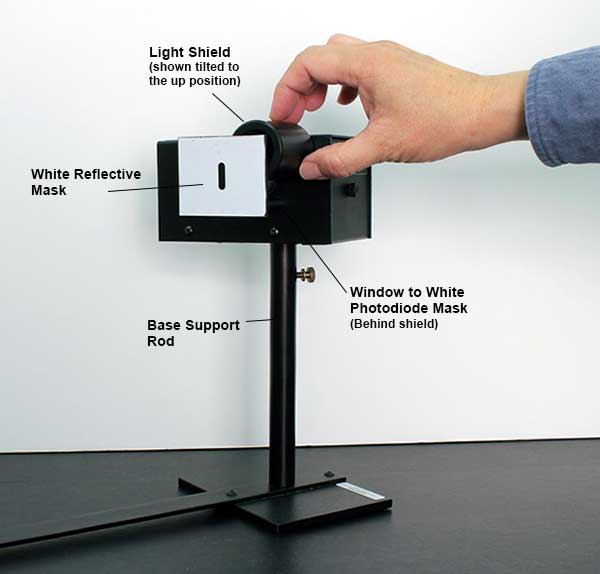 Connect the digital voltmeter (DVM) to the “Output” terminals of the photodiode. Select the 2 V or 20 V range on the meter. Press the “Push to Zero” button on the side panel of the photodiode housing to short out any accumulated charge on the electronics. Note that the output will shift in the absence of light on the photodiode. Record the photodiode output voltage on the DVM. This voltage is a direct measure of the stopping potential. Use the green and yellow filters for the green and yellow mercury light. These filters block higher frequencies and eliminate ambient room light. In higher diffraction orders, they also block the ultraviolet light that falls on top of the yellow and green lines. PROCEDURE PART 1: DEPENDENCE OF THE STOPPING POTENTIAL ON THE INTENSITY OF LIGHT Adjust the angle of the photodiode-housing assembly so that the green line falls on the window of the photodiode. Install the green filter and the round light shield. Install the variable transmission filter on the collimator over the green filter such that the light passes through the section marked 100%. Record the photodiode output voltage reading on the DVM. Also determine the approximate recharge time after the discharge button has been pressed and released. Repeat steps 1 – 3 for the other four transmission percentages, as well as for the ultraviolet light in second order. Plot a graph of the stopping potential as a function of intensity. PROCEDURE PART 2: DEPENDENCE OF THE STOPPING POTENTIAL ON THE FREQUENCY OF LIGHT You can see five colors in the mercury light spectrum. The diffraction grating has two usable orders for deflection on one side of the center. Adjust the photodiode-housing assembly so that only one color from the first-order diffraction pattern on one side of the center falls on the collimator. For each color in the first order, record the photodiode output voltage reading on the DVM. For each color in the second order, record the photodiode output voltage reading on the DVM. Plot a graph of the stopping potential as a function of frequency, and determine the slope and the \(y\)-intercept of the graph. From this data, calculate \(W_0\) and \(h\). Compare this value of \(h\) with that provided in the “Introduction” section of this experiment. 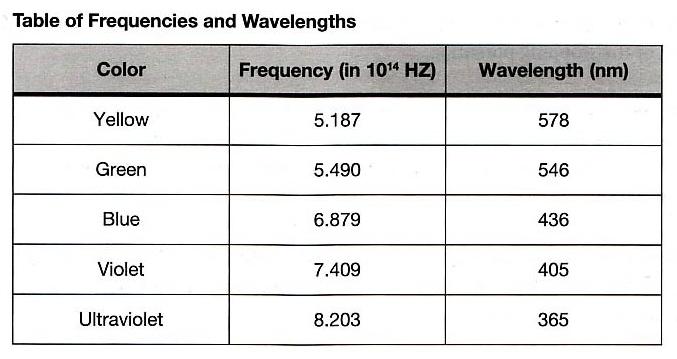 Procedure Part 1: Photodiode output voltage reading for 100% transmission = Approximate recharge time for 100% transmission = Photodiode output voltage reading for 80% transmission = Approximate recharge time for 80% transmission = Photodiode output voltage reading for 60% transmission = Approximate recharge time for 60% transmission = Photodiode output voltage reading for 40% transmission = Approximate recharge time for 40% transmission = Photodiode output voltage reading for 20% transmission = Approximate recharge time for 20% transmission = Photodiode output voltage reading for ultraviolet light = Approximate recharge time for ultraviolet light = Plot the graph of stopping potential as a function of intensity using one sheet of graph paper at the end of this workbook. Remember to label the axes and title the graph. Procedure Part 2: First-order diffraction pattern on one side of the center: Photodiode output voltage reading for yellow light = Photodiode output voltage reading for green light = Photodiode output voltage reading for blue light = Photodiode output voltage reading for violet light = Second-order diffraction pattern on the other side of the center: Plot the graph of stopping potential as a function of frequency using one sheet of graph paper at the end of this workbook. Remember to label the axes and title the graph. Slope of graph = \(y\)-intercept of graph = \(W_0\) = \(h\) = Percentage difference between experimental and accepted values of \(h\) =  {:instructional:lab_manuals:physics:6c:experiment_7:photo_graph_2.jpg|}}  Table of Contents- 18.1. Background
- 18.2. Experimental Equipment
- 18.3. Pre-Lab Preparation
- 18.4. Experimental Procedure
- 18.5.1. Analysis hints:
Related Topics- Previous: 17. Lenses and Optical Instruments
- Next: 19. PN-Junction
Quick search18. the photo-electric effect ¶, 18.1. background ¶. The experiment serves to demonstrate the photoelectric effect, for which Einstein was awarded a Nobel prize, and in the process determine Planck’s constant, \(h\) . The photoelectric effect is the process whereby a photon of energy \(E=h\nu\) , incident on the surface of a conductor, transfers its energy to one of the electrons of an atom. If the energy is sufficient, the electron can not only escape from the material, but do so with a certain amount of kinetic energy. If the electron is in the highest available energy state within the conductor, the least amount of energy, \(\phi\) , is needed to free it, and it will escape with the maximum kinetic energy. \(\phi\) is called the work function of the conducting material.  Fig. 18.1 Schematic of the photo-electric effect experiment. A photon hits the conducting anode and knocks out an electron. All electrons that have sufficient kinetic energy to reach the cathode produce an electric current. The adjustable stopping voltage determines the minimal kinetic energy the electrons need. ¶ If the conductor forms the anode of a phototube, as shown in Fig. 18.1 , no electrons will reach the cathode if the potential difference ( retarding potential ) between the anode and cathode is adjusted to the minimum value necessary to stop the fastest electrons. The loss of kinetic energy is then balanced by the gain of potential energy, \(e V_s\) , and the energy equation is given by where \(V_s\) is called the stopping potential . The threshold frequency , \(\nu_0\) , is the minimum photon frequency capable of eliciting the photoelectric effect. It is obtained by setting \(V_s = 0\) in equation (18.1) : In the experiment, a monochromator is used to isolate a number of different wavelengths from a mercury lamp, and for each the stopping potential is determined. From the data, \(h\) , \(\phi\) , and \(\nu_0\) may be determined. 18.2. Experimental Equipment ¶The setup for this experiment is shown in Fig. 18.2 . It consists of a mercury lamp, a monochromator, the photocell with a control for the stopping voltage and a current amplifier with a zero adjust for the photo-current measurement. Be careful with the mercury lamp. It is made of quartz, emits UV and gets very hot .  Fig. 18.2 Overview of the main equipment parts of the photo-electric effect experiments. ¶ You will also need two additional digital multimeters to measure the stopping voltage and the photo current. A close-up of the instrument is shown in Fig. 18.3 .  Fig. 18.3 The monochromator and the instrument containing the photocell. The instrument can be removed when the sleeve is pushed toward the monochromator. Then the exit slit can be viewed to select which Hg spectral line is used. Then you remove the instrument make sure that it is either turned off or that the stopping voltage is at a maximum (know turned CW as far as it goes) ¶ The wavelength can be adjusted by turning the knob at the side if the monochromator (this rotates the grating inside the monochromator). An arrow indicates the direction towards shorter \(\lambda\) . 18.3. Pre-Lab Preparation ¶Assume you have determined that the stopping voltage for the yellow light is 0.5V and it is 1.5V for the ultraviolet light. Determine the value of hc? The wavelengths of the mercury spectrum can be found in the manual. Express the results using the unit of ev*nm. Prepare the data file formats for each wavelength and store the wavelength as a parameter in the header section. Prepare a python script to plot your data while you are taking them. This makes sure that you have good quality data. 18.4. Experimental Procedure ¶DAMAGE COULD RESULT IF THE UNIT IS TURNED ON WHILE THE PHOTOELECTRIC CELL IS EXPOSED TO ROOM LIGHT OR INTENSE LIGHT Turn on the mercury lamp and wait for about 5 minutes until it reaches its full intensity. Connect one of the digital multimeters to measure the retarding potential and set it to the 20 V DC range. Connect the second multimeter to measure the photoelectric cell current (thereby duplicating the reading on the less accurate built-in milliammeter) and set it to the 2 mA DC range. By turning the wavelength control on the side of the monochromator, different spectral lines will become visible at the exit slit. Those to be used are: yellow (578 nm), green (546 nm), blue (436 nm) violet (405) and ultra-violet (365 nm). The violet appears relatively faint and the ultra-violet is, of course, invisible. Adjust the wavelength control until the yellow line is visible. Place the photoelectric cell on the stand forming a light tight seal with the monochromator. Turn the “voltage adjust” control fully clockwise (maximum retarding potential) and then switch on the power switch. Cover the entrance slit of the monochromator to prevent light entering and adjust the “zero adjust” until zero current is obtained. This is a very delicate adjustment and you may not be able to obtain a precise zero. Let the light back into the system. Turn the “voltage adjust” control in a counter-clockwise direction, thereby reducing the retarding potential, until a current of about 1 mA is registered. Now adjust the wavelength control until the current is maximum. You have now optimized the monochromator for 578 nm. Turn the “voltage adjust” control fully clockwise (maximum retarding potential) Before recording the “stopping potential”, double check the zero setting, blocking the light as before. After you let the light back into the system you will notice a small negative current which is normal. Try to figure out where this current is coming from. Measure the current as a function of the retarding potential by reducing the retarding voltage first in step of 0.5 V and as soon as you see a noticeable change in the photo current decrease the voltage steps in such a way that you have about 10 measurements in the ‘knee region’ and data up to a current of about 0.5 mA. You should end up with about 15 - 20 data points for each color. Having completed this wavelength, turn the “voltage adjust” control fully clockwise and remove the photoelectric cell so that you can adjust the monochromator for the next spectral line. Repeat the above procedures for each line in turn. The ultra- violet line will have to be found by adjusting the wavelength control beyond the position for the violet line until a current is registered. Keep adjusting the “voltage adjust” control to prevent the current reading from going off-scale while seeking the maximum current. 18.5. Analysis ¶The goal of the analysis is to carefully determine the minimal retarding voltage from which one can determine kinetic energy of the ejected photo-electrons.  Fig. 18.4 Typical current vs. stopping voltage ( \(V_s\) ) curve for the UV line. Note the ‘knee` and the two limiting lines fitted to determine the range of values for the stopping voltage. ¶ Each data set taken for a specific wavelength needs to be analyzed separately and the general method is as follows: Plot the measured current, \(I\) as a function of the stopping potential \(V_s\) . A typical example is shown in Fig. 18.4 . Note that the current increases smoothly and it is not obvious how to determine the exact value of the minimal value of \(V_s\) . A possibility is so fit a line to the data where \(V_s\) is large and the current changes only lightly. In the area where you see the ‘knee’ in the curve select a second range of points where the current starts to rise (typically a current increase of 200 - 400 \(\mu A\) works well). Fit a second line to these data and calculate the intersection of the two lines. The value for \(V_s\) at the intersection can then be interpreted as the minimal stopping potential to prevent electrons from reaching the anode and the potential energy of the electrons when they just reach the anode corresponds to their kinetic energy when the were just ejected from the cathode. In order to get an estimate of the uncertainty use limiting cases in your selection of data points to determine these two lines (see Fig. 18.4 ) Use the voltages and their uncertainties determined in the previous step to determine \(h\) and \(\phi\) by fitting a line to the measured electron energies as a function of the frequency of the light. Why to you measure a current in the opposite direction of the photo current when \(V_s\) is maximal and you carefully zeroed the current measurement. 18.5.1. Analysis hints: ¶How to fit a line to a subset of data of \(I\) and \(V\) ?: Select the data using: Here ir contains the information on the data where the current I is between -0.1 and 0.3. The fit results are stored in l1. The most important are: l1.slope and l1.offset and their uncertainties, l1.sigma_offset and lq.sigma_slope . For further information check the general documentation. If you prefer to select data using the voltage you would do: Here vh contains the information on the data where the voltage V is between 1.5 and 3.5 and the fit results are stored in l2. Instead of using B.in_between you can also do: which is what B.in_between actually does. The Photoelectric Effect - Carbon Dioxide
- Exploration
- Sample Problems
- Simulated Experiment
- Links to Literature
- Instructions
Getting Started Trial | Metal | Voltage (V) | Current (pA) | Frequency (Hz) | Wavelength (nm) | Photon DensityVoltage (v).  Name: | The Photoelectric Effect | Version/Date: | Version 3.1, 30 Aug 2021 | Authors: | Marc-Olivier Lajeunesse, Tyler deBoon, Dr. Rob MacDonald, Andrew Martin, Dr. Brian Martin, Dr. Peter Mahaffy, | Contact: | Visit for contact information | | --> | Item added to your cartDemonstration of the photoelectric effect. Featured ProductsIn 1905 Albert Einstein had his miracle year, publishing 5 papers, including the Special Theory of Relativity, the Mathematical Description of Brownian Motion, and the E=mc 2 formula. One of these papers was titled “On a Heuristic Point of View about the Creation and Conversion of Light.” [Ref 1] In section 8 of this paper he develops a mathematic model that describes how light creates cathode rays. What was heuristic was that he described light as “energy quanta” (photons) for the first time but what was worth a Nobel Prize, was that he was correct. 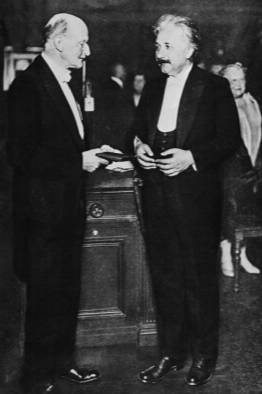 Figure 1a Max Planck presents Einstein with the 1929 Planck Medal for extraordinary achievements in theoretical physics. 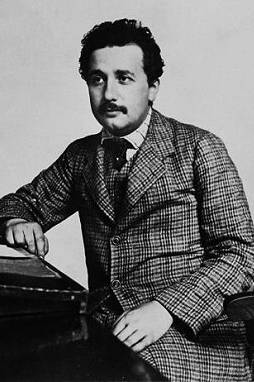 Figure 1b Albert Einstein c.1905 at the patent office desk where he worked as a third-class clerk, and occasionally worked on his “miracle” papers. It is quite easy to demonstrate the photoelectric effect with an electroscope and a short wave UV-C lamp . By placing a negative charge on the electroscope, and shining the short wave UV light on top, it will discharge. Short wave UV is usually blocked by glass, but visible light is not, thus a pane of glass can be used to show that it is not just regular light that is causing the discharge. It helps that on the top of the electroscope there is a zinc plate which has been scrubbed with steel wool. This removes the zinc oxide layer and makes it more sensitive to the light. Also note that if the experiment is tried with a positive charge, for example with a piece of glass rubbed with silk, it will fail to discharge. This is because the photoelectric effect is only for electrons and will only work on negative charges because they are at the surface, but the positive charges are held deep in the nucleus by the strong force. In fact, the photoelectric effect is a great way to identify positive vs. negative charge. 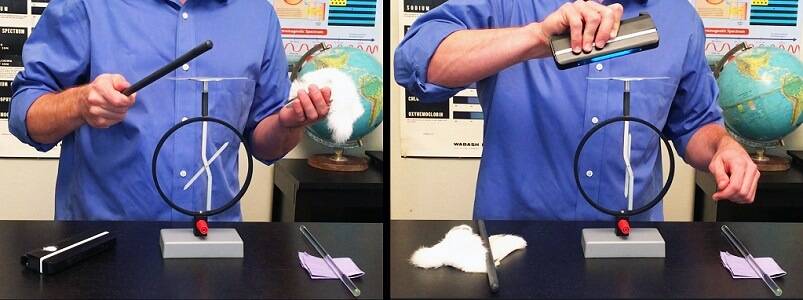 Figure 2. The electroscope is discharged by shining short wave ultraviolet light upon it. With any form of visible light, you will not get the electroscope to discharge. It does not matter how bright the light is, even lasers will fail. It takes ultraviolet, specifically the short wave UV-C, because even black lights are not energetic enough to liberate the electrons. The thing with light is, the shorter wavelength the more energy it has, and this was already well-known and given by Max Planck’s formula E = h f , but it was the idea that light hits one electron at a time individual pieces of light, not as waves, that won Einstein the Nobel Prize. ONE particle of light hitting ONE electron at a time. Einstein called this particle a quantum of light, meaning that it is a discrete exchange of energy. We now call this a PHOTON. Albert Einstein took Max Planck’s formula for the quantization of energy in black bodies and extended it to describe light. This implied that light delivered its energy in bundles of E=hf. This was a new and heuristic idea, but it explained the photoelectric effect. KE is the energy of the escaping electrons and W is the energy required to liberate them from the metal. 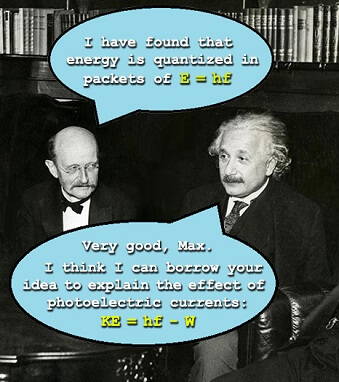 Figure 3. Max and Albert have a chat The Phet Simulation of the photoelectric effect is a great way to engage students with the details of this modern physics concept. It is based on the experiments of Robert A. Millikan who proved Einstein’s perspective by experiment. It is also possible to demonstrate the photoelectric effect with a small neon bulb. These will turn on at about 70 volts. Hook one to a high voltage source and then dial it back until it is just about to turn on. Now it will be so sensitive, that just a little extra energy will make the electrons jump and conduct. This will NOT work for red and green light. It must be blue, or ultraviolet. That’s right, blue light ACTUALLY DOES have more energy than red and green light. This is because light travels as photons, and the shorter the wavelength of light, the more energy per photon. 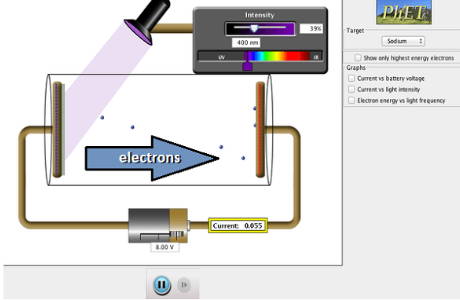 Figure 4. Screenshot of the PhET simulator 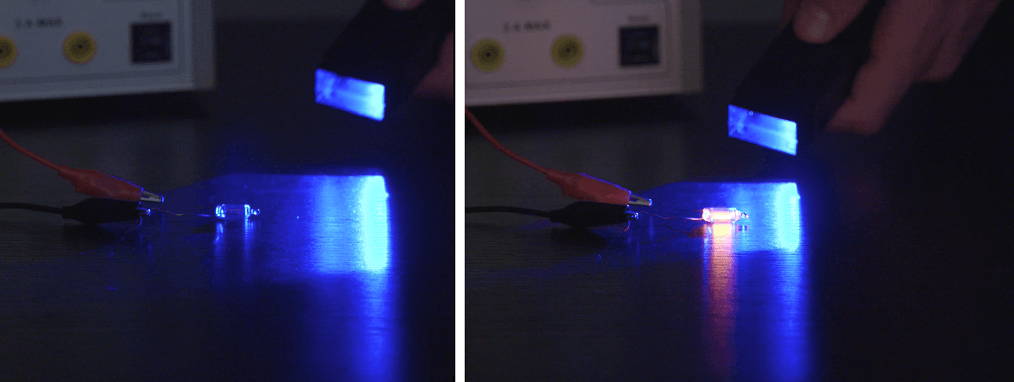 Figure 5. The energy of blue light can cause electron conduction in a small neon bulb. The bulb stays on because plasma conducts better than rarefied neon. Reference: http://hermes.ffn.ub.es/luisnavarro/nuevo_maletin/Einstein_1905_heuristic.pdf February 20, 2018 James Lincoln - Share on Facebook
- Tweet on Twitter
- Pin on Pinterest
Leave a commentPlease note, comments need to be approved before they are published. Customer ServiceMon-Fri: 8:30am-5pm EST 1556 Woodland Dr E Saline, MI 48176 "We strive to respond with consistency, fairness, grace, and intelligence. Each customer deserves special attention." — C. Peter Rea, President Back Order DetailsItems can be ordered now, and we'll deliver when available. Create A QuoteWe made it easier than ever to create a quote for budget approvals or to facilitate a purchase order requisition! - Simply click the "Create A Quote" button and you will be taken through the process.
- Filling out your billing and shipping information and selecting a shipping option will calculate your shipping costs.
- After completing the process, your quote will be automatically saved to your user account.
- At any point, you will be able to edit, print, share or convert a quote into and order from your user account.
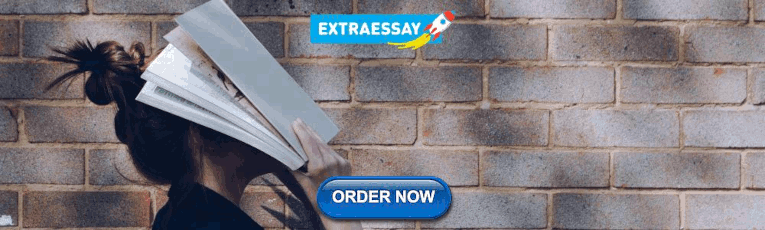 WHAT TYPE OF LAB?From beakers and test tubes to safety goggles and sensors — you'll find a variety of products in our Lab Builders. - Choosing a selection results in a full page refresh.
SPARKvue 4.10 Reimagined Data Collection. Free Trial Photoelectric Effect Experiment • EX-5549A- Thermodynamics
- Electromagnetism
- Waves & Optics
Sensors & DataloggingCurriculum & bundles, lab apparatus & supplies, stem sense solutions, product guides.  Explore Our 2024 Catalogs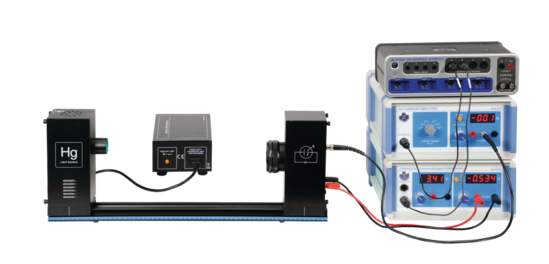 Complete photoelectric effect experiment includes experiment files and the photoelectric effect system; complete solution designed for use with PASCO Capstone Software. - 1x Basic Photoelectric Effect Apparatus
- 1x DC Current Amplifier
- 1x DC Power Supply I (Constant Voltage)
- 1x Cables for 850 Interface
Product SummaryUse the 850 Universal Interface and PASCO Capstone to collect and analyze data. Both the picoammeter and the power supply for the stopping voltage have sensor ports on the front that connect to the analog sensor ports of the 850 Universal Interface. PASCO Capstone automatically recognizes these instruments and can read the current and the voltage. During the experiment, each time a different filter is applied, the user clicks “Keep” in PASCO Capstone and the value of the stopping voltage for that frequency is recorded and automatically graphed vs. frequency. - Connects to the 850 Universal Interface for data collection in PASCO Capstone
- Find Planck’s Constant to within 5%
- Verify that stopping voltage is independent of intensity
- Find characteristics of the photodiode
What's Included- 1x Basic Photoelectric Effect Apparatus (SE-6614)
- 1x DC Current Amplifier (SE-6621)
- 1x DC Power Supply I (Constant Voltage) (SE-6615)
Data Collection SoftwareThis product requires PASCO software for data collection and analysis. We recommend the following option(s). For more information on which is right for your classroom, see our Software Comparison: SPARKvue vs. Capstone » Interface RequiredThis product requires a PASCO Interface to connect to your computer or device. We recommend the following option(s). For a breakdown of features, capabilities, and additional options, see our Interface Comparison Guide » - 550 Universal Interface
- 850 Universal Interface
ExperimentsExperiment LibraryPerform the following experiments and more with the Photoelectric Effect Experiment. Visit PASCO's Experiment Library to view more activities. Photoelectric EffectThe photoelectric effect is the emission of electrons from the surface of a metal when electromagnetic radiation (such as visible or ultraviolet light) shines on the metal. In this lab, you will study the effect varying the light... Support DocumentsManuals | | | English - 2.14 MB |
Product Videos Photoelectric System - EX-5549A Photoelectric Effect SystemRelated products & accessories. 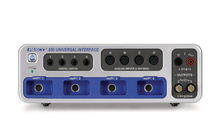 Science simulations Photoelectric Effect ExperimentPhotoelectric effect. The photoelectric effect is the phenomenon that the electrons pop out when a light beam incident on a metal surface. It can be thought that the energy of light is transformed into the form of electrical energy. However, electrons will only be emitted when the light is incident on the metal at a certain frequency or higher. The minimum photon energy at which electrons can stick out is the 'work function.' Usually, alkali metals such as sodium(Na) are often used in photoelectric effect experiments. This is because alkali metals' work function is small, so it is easy to cause a photoelectric effect even with low-frequency visible light. 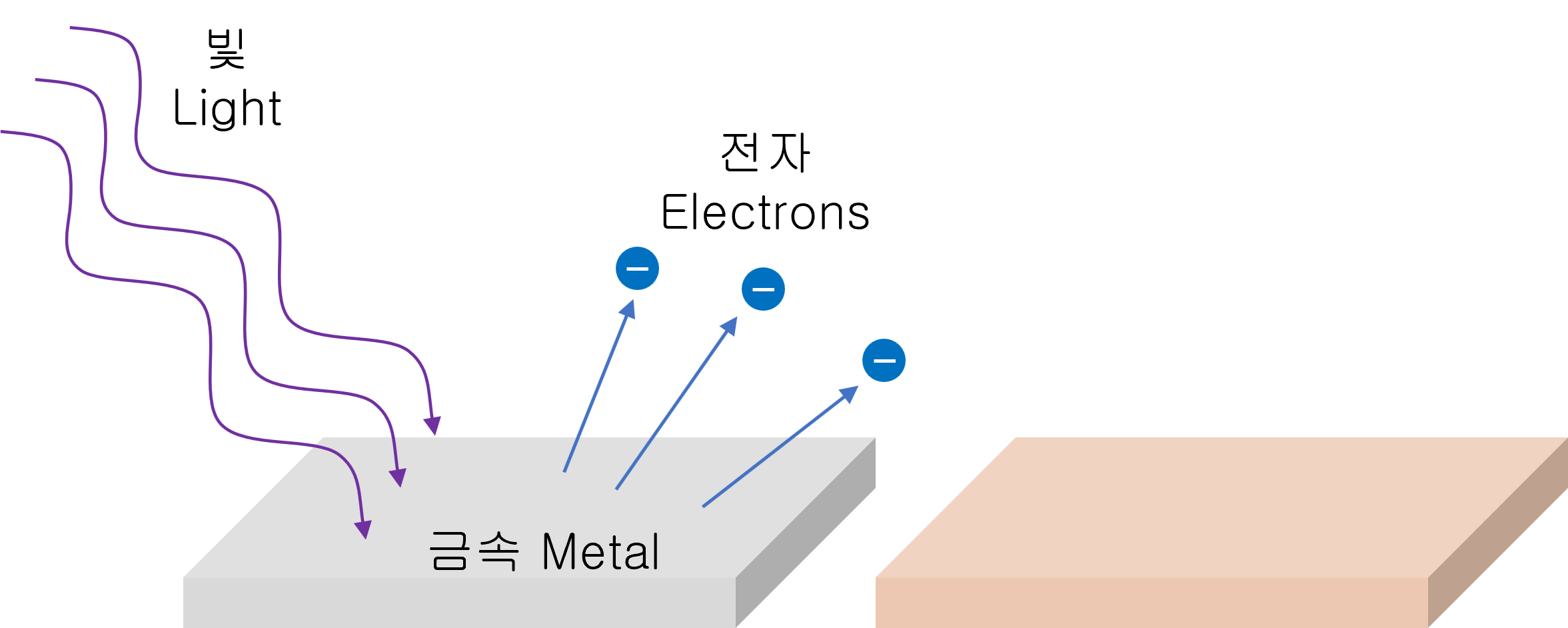 Energy of one photonLight(electromagnetic waves) is both a wave and a particle. If light(photons) is at the same frequency, the energy carried by the light(photons) is the same. Light moves like individual particles and half particles do not exist. The amount of energy carried by a single light particle(photon) is: \[ E = h\nu \] h is Planck's constant(6.626×10 -34 J·s), and ν (nu) is the frequency of light(s -1 ). If the speed of light is c (299,792,458 m/s), \[ \nu =\frac { c }{ \lambda } \] You can also use the energy size of a photon as : \[ E=\frac { hc }{ \lambda } \] λ(lambda) is the wavelength of light in meters Kinetic energy of photoelectron and 'stopping Voltage'In a photoelectric effect experiment device such as the simulation above, when monochromatic light shines on a metal plane, electrons are released due to the metal plate's photoelectric effect. At this time, if a voltage is applied between the anode and the cathode to interfere with the emitted electrons' movement, the kinetic energy of the emitted electrons decreases, and eventually, the electrons will not be released. This voltage is called the 'stopping voltage.' In other words, the maximum kinetic energy K max of the electrons released without stopping voltage is equal to the stopping voltage multiplied by the amount of electron charge. \[ { K }_{ max }\, =\, eV \] Also, the metal surface itself may interfere with the emission of electrons. This energy is the 'work function.'Therefore, if the stopping voltage is not applied, We can calculate the emitted electrons' kinetic energy. \[ { K }_{ max }\, =\, h\nu \, -\, W \] When stopping voltage is applied, the maximum kinetic energy of emitted electronsWhen the stopping voltage is applied, the maximum kinetic energy K max of the emitted electron is calculated as follows. \[ { K }_{ max }\, =\, h\nu \, - \, W \, - \, (Energy loss due to stopping voltage) \] Convert unit J of energy to eVIn terms of the photoelectric effect, it is convenient to change the unit of energy to eV.1 eV is the amount of energy when one electron is accelerated at a potential of 1V. \[ 1eV \, = \, 1.6×{ 10 }^{ -19 }J \] Future energy source, the sunThis photoelectric effect becomes the basic concept of solar power generation. In other words, when light is emitted above a certain frequency, electrons pop out immediately (no delay in time). If these electrons are allowed to run through electrical circuits, then solar power is generated. Solar cells are semiconductors made from silicon (Si) in the sand. When sunlight shines on a solar cell, the electrons move and flow along the wires, and the flow of electrons creates electrical energy. 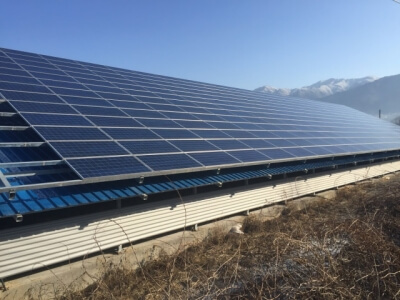 Solar cells are made by adding tiny amounts of phosphorus and boron to each side of thin silicon plates, which are commonly used as semiconductors. Most commercially available silicon solar cells can convert 15 to 25 percent of the energy in sunlight. The output voltage of one solar panel is only about 0.6V. So we connect several plates to increase the output voltage and current. PhotoElectric Effect Simulation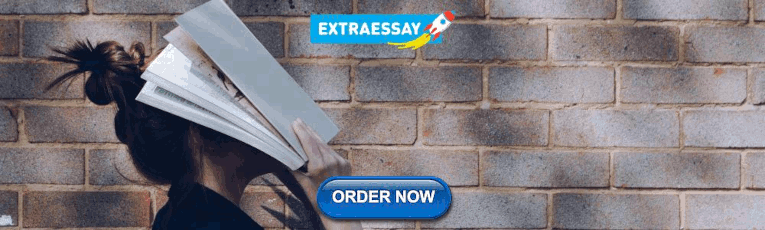 |
IMAGES
VIDEO
COMMENTS
See how light knocks electrons off a metal target, and recreate the experiment that spawned the field of quantum mechanics.
The photoelectric effect was able to be observed from the first part of the experiment due to the backing voltage of 0V and tube current of 0μA. In the second part, the planck's constant of 1 × 10 − 28 J was able to determine from the graph of stopping or backing voltage versus frequency and in comparison, with the accepted value of the ...
As the first experiment which demonstrated the quantum theory of energy levels, photoelectric effect experiment is of great historical importance. The important observations on Photoelectric effect which demand quantum theory for its explanation are: The Photoelectric effect is an instantaneous phenomenon.
Experiment 6 - The Photoelectric Effect. APPARATUS. INTRODUCTION. The energy quantization of electromagnetic radiation in general, and of light in particular, is expressed in the famous relation. where E E is the energy of the radiation, f f is its frequency, and h h is Planck's constant (6.63×10 -34 Js). The notion of light quantization was ...
18. The Photo-Electric Effect¶ 18.1. Background¶. The experiment serves to demonstrate the photoelectric effect, for which Einstein was awarded a Nobel prize, and in the process determine Planck's constant, \(h\). The photoelectric effect is the process whereby a photon of energy \(E=h\nu\), incident on the surface of a conductor, transfers its energy to one of the electrons of an atom.
This effect was named the photoelectric effect, and the emitted electrons called photoelectrons. To explain the effect, a mechanism was proposed by which the energy in the ... the experiment and findings. The lab report must not exceed 6 pages of an electronic document in the format PDF or Word, or any other format that permits commenting by an ...
Photoelectric effect experiment equipment. (Image courtesy of MIT Junior Lab staff.) The maximum kinetic energy of electrons ejected from a metal surface by monochromatic light is measured for several wavelengths. The value of Planck's constant, h, is derived by an analysis of the data in the light of Einstein theory of the photoelectric effect.
The Photoelectric Effect. MIT Department of Physics. The objective of this experiment is to demonstrate the quantization of energy in electromagnetic waves and to determine Planck's constant h. You will measure the maximum kinetic energy of electrons ejected by the photoelectric effect from an alkali metal surface as a function of frequency.
the photoelectric effect and Einstein's explanation. Instructor's Expectations During the lab session students • Assemble the apparatus; • Make a sketch, or a photo, or use any available source to present the working setup in the lab report with main parts of the apparatus linked to the drawing in Fig. 2; • Perform exercises detailed ...
PHY 312: Advanced Lab Photoelectric effect 1 Background A photon of frequency ν carries energy hν, where h is Planck's constant. If such ... The light source for this experiment is a halogen lamp, which can be approx-imated as a black body with maximum intensity around λ = 550nm. Light from
Lab 2: The Photoelectric Effect. You have observed the diffraction and interference patters produced by single and multiple slits. One can predict the positions of the maxima and minima in these patterns by assuming that light is an EM wave. Where crests meet crests and troughs meet troughs we predict and observe maxima or bright regions, and ...
Apparatus. The photoelectric x- effect in this e periment occurs at the cathode of an IP39 phototube. The phototube is constructed as shown in Figure 2. The IP39 phototube is designed to function as a diode i- where light, inc dent on the tube, illuminates the cathode and-emission causes photo of electrons. The central wire (anode) is shielded ...
Lab Reports for EIO and Prelab Questions for Ell are due at the beginning of this lab. Objectives Understand how ejection of photoelectrons from a metal surface depends on the frequency and intensity of incident light. Measure Planck's constant and the work function for the cathode material. Theoryl The photoelectric effect is one of several ...
Experiment 10: The Photoelectric Effect April 28, 2009 In the late 1800's, many thought that all the main principles and law of nature had been discovered. However, there were still a few puzzling discrepancies. One of these is the experimental data on the emission and absorption of electromagnetic radiation from a blackbody.
This DLO is a virtual reproduction of Millikan's photoelectric experiment. Use either the input box or the slider bar to adjust the wavelength of incoming photons. Adjust the photon density slider to change the photon density or the number of photons of incoming light. The Voltage slider will create an electric field to oppose the electrons ...
THE PHOTOELECTRIC EFFECT AND PLANCK'S CONSTANT. White light that is passed through various filters illuminates the photoelectric surface of a phototube causing photoelectrons to be emitted with energies dependent upon the energy of the light hitting the surface. The electrons then flow through the phototube to the collector and constitute a ...
See how light knocks electrons off a metal target, and recreate the experiment that spawned the field of quantum mechanics.
The photoelectric effect is the emission of electrons from the surface of a metal when electromagnetic radiation (such as visible or ultraviolet light) shines on the metal. In this lab, you will study the effect varying the light intensity and frequency has on the energy of the emitted electrons and the magnitude of the photo-current. You will also determine Planck's constant.
Demonstrating the photoelectric effect is as easy as using an electroscope and a few common lab materials. In this post, watch physics teacher James Lincoln demonstrate the photoelectric effect. ... Also note that if the experiment is tried with a positive charge, for example with a piece of glass rubbed with silk, it will fail to discharge. ...
Photoelectric Effect Experiment • EX-5549A. Photoelectric Effect Experiment. •. EX-5549A. For the typical sample data shown, the graph of Stopping Voltage vs. Frequency gives a slope of 4.2 x 10 V·s. This results in a value for Planck's Constant of 6.7 x 10 J·s, which is 1.3% above the accepted value. Graph generated using PASCO ...
In a photoelectric effect experiment device such as the simulation above, when monochromatic light shines on a metal plane, electrons are released due to the metal plate's photoelectric effect. At this time, if a voltage is applied between the anode and the cathode to interfere with the emitted electrons' movement, the kinetic energy of the ...
PhotoElectric Effect Simulation. select the desired cathode metal from guipanel and adjust wavelength, intensity of light and potential of source to see their effect on photoelectric current. There is also a graph showing variation of current with voltage of source. Controls. Drag the sliders on battery to change its voltage. Drag over the ...